Introduction
Materials and Methods
포트시험
토양 화학성 분석
식물체 특성 분석
통계분석
Results and Discussion
목재 바이오차 시용에 따른 토양 화학성 변화
목재 바이오차 시용에 따른 상추 생산성 변화
목재 바이오차 시용에 따른 상추 양분 흡수량 변화
Conclusions
Introduction
바이오차 (biochar)는 다양한 바이오매스를 제한된 산소 조건에서 열분해하여 제조한 고탄소 화합물이다 (Woolf et al., 2010; Park et al., 2021). 바이오차 제조에 가장 널리 활용되고 있는 원료는 목재이며, 최근 왕겨, 볏짚 등의 농업부산물 또한 원료로서 활용가능성이 대두되고 있다. 바이오차는 제조 중 열분해 과정을 통해 구조적으로 난분해성 탄소화합물로 전환되기 때문에, 이를 토양에 투입할 경우 탄소 격리 (carbon sequestration) 효과를 기대할 수 있다 (Lehmann et al., 2006; Rumpel et al., 2006; Yin et al., 2014; Budai et al., 2016). 또한, 바이오차의 물리적 (다공성, 비표면적 등) 및 화학적 특성 (pH, 양이온교환용량 등)으로 인해 토양개량제로서 활용가치가 높다고 평가받고 있다. 이에 따라 최근 토양 질 개선 및 지구온난화 완화 등을 위해 바이오차를 활용한 탄소농업 (carbon farming)이 주목받고 있다 (Nogues et al., 2023).
토양의 물리 ‧ 화학 ‧ 생물학적 특성에 대한 바이오차의 시용효과는 다양한 연구를 통해 보고되고 있다 (Lehmann et al., 2011; Singh et al., 2022). 예를 들어, 바이오차를 구성하는 미세 공극에 의한 토양의 비표면적 증가로 토양 수분 보유력이 향상되며 (Razzaghi et al., 2020; Roy et al., 2022), 양이온교환용량 증가에 따라 토양의 양분 보유능이 증대되는 효과가 있다 (Hammes and Schmidt, 2009; Masud et al., 2020). 이 외에도, 바이오차 시용에 의한 산성토양 개선 및 미생물 활성 증가 (Paz-Ferreiro et al., 2014; Chen et al., 2017), 중금속 부동화 (Qasim et al., 2021; Chen et al., 2023) 및 작물의 생산량 증대 (Jaiswal et al., 2017; Lee et al., 2022; Edussuriya et al., 2023)와 같은 긍정적 효과가 보고되고 있다.
하지만, 바이오차의 특성은 원료 및 열분해 조건 등에 따라 다르게 나타나난다 (Mukherjee and Zimmerman, 2013; Kim et al., 2022a). 뿐만 아니라 경작지 토양의 특성과 대상작물 등에 따라 적정 시용량이 크게 달라질 수 있다. 최근 국내에서는 바이오차의 처리 수준에 따른 토양특성 및 작물생산성 차이에 대한 연구가 수행되고 있지만 (Kang et al., 2016; Kim et al., 2022b), 해외 연구사례에 비해 매우 미흡한 실정이다. Yi et al. (2019)의 연구에 따르면, 밭토양에서 상추 재배 시 목재 바이오차의 최적 시용량은 2 Mg ha-1으로 나타났다. 하지만 이는 단기간 연구결과로, 바이오차 연용 시비 시 시비효과 및 최적 시용량은 상이할 것으로 사료된다. 따라서, 본 연구에서는 목재 바이오차의 연용 처리를 통해 밭 토양의 화학적 특성과 상추 수확량 및 품질에 미치는 영향에 대해 알아보고 이를 근거로 적정 시용량을 산출하고자 수행하였다.
Materials and Methods
포트시험
본 연구에 사용된 목재 바이오차는 ㈜한국남동발전에서 목재펠렛을 연소한 뒤 발생한 화력발전 부산물로 유기농업자재 인증을 받은 제품을 사용하였으며, 특성은 Table 1과 같다. 대상작물로 적치마상추 (Lactuca sativa L. var. Red skirt)를 선정하였으며, 상추 재식거리를 고려하여 Wagner pot (1/5,000 a-1)에 하나의 상추모종을 정식하였다. 상추 정식 10일 전 무기질 비료와 바이오차를 토양에 처리하고 균질화하였다. 목재 바이오차 처리수준은 Yi et al. (2019)의 선행연구 결과를 근거로 바이오차 적정 시용량 (2 Mg ha-1)을 기준으로 이의 0% (0 Mg ha-1, Control), 50% (1 Mg ha-1, WB100), 100% (2 Mg ha-1, WB200), 200% (4 Mg ha-1, WB400) 및 400% (8 Mg ha-1, WB800)에 해당되는 양을 Wagner pot (1/5,000 a-1)에 면적대비로 산출하여, 각각 3반복으로 구성하였다. 또한, 무기질 비료 처리량은 작물별 비료사용처방기준 (RDA, 2019)에 근거하여 시험토양의 화학적 특성 분석 결과 (Table 2)를 토대로 모든 처리구에 검정시비량 (N–P2O5–K2O = 0.12–0.29–0.07 Mg ha-1)으로 전량 밑거름 시비하였다. 포트시험은 경상국립대학교 교내 야외농장에서 수행되었으며, 1차 재배는 2023년 5월 19일부터 7월 13일, 2차 재배는 2023년 8월 31일부터 10월 31일까지 약 60일간 수행하였다. 2차 재배기간 중 기온 및 날씨의 영향으로 상추의 발달 및 생장이 1차 재배 때보다 더디게 나타났다. 두 작기 동안 바이오차 및 무기질비료를 연용 시비하였으며, 재배 후 상추의 지상부만 수확하여 생중량 및 양분 흡수량을 분석하였다.
Table 1.
Chemical properties of wood-derived biochar used in this study.
pH (1:10CaCl2) |
EC (dS m-1) |
Ash (%) |
C (%) |
H (%) |
O (%) | O/C1 | H/C1 |
8.4 | 10.9 | 13.1 | 76.3 | 1.2 | 22.1 | 0.22 | 0.19 |
Table 2.
Chemical properties of initial soil used in this pot experiment.
pH (1:5w) |
EC (dS m-1) |
OM (g kg-1) |
T-N (%) |
Av. P2O5 (mg kg-1) |
CEC (cmolc kg-1) |
Exch. K (cmolc kg-1) |
Exch. Ca (cmolc kg-1) |
Exch. Mg (cmolc kg-1) |
6.4 | 0.54 | 15.4 | 0.11 | 55.0 | 13.6 | 0.6 | 9.4 | 3.5 |
토양 화학성 분석
토양의 화학적 특성 분석을 위해 재배시험 전후로 각 처리구의 표토 (0 - 15 cm)를 채취하여 풍건 및 2 mm 체질 후 사용하였다. 토양 pH와 전기전도도 (electrical conductivity, EC)는 토양과 증류수를 1:5 중량비로 혼합 후 교반하여 각각 pH meter (Orion Star 2-Star, Thermo Scientific, USA)와 EC meter (Orion 3-Star, Thermo Scientific, USA)로 측정하였으며, EC는 희석배수를 곱하여 산출하였다. 유효인산은 Lancaster법 (NIAST, 2000)에 따라 추출 후 UV/Vis spectrophotometer (CARY 50 CONC, Varian, USA)를 이용하여 분석하였다. 총 질소 (T-N)는 Kjeldahl법 (Bremner, 1996)에 따라 정량하였으며, 유기물은 Tyurin법 (Nelson and Sommers, 1996)으로 측정하였다. 양이온교환용량 (cation exchange capacity, CEC)과 교환성양이온 (exchangeable cation, Exch. Cation)은 1 N NH4OAc (pH 7.0) 용액으로 추출 후 각각 Kjeldahl (K-355, BÜCHI, Switzerland)과 ICP-OES (BRE731400, Thermo Scientific, USA)로 분석하였다.
식물체 특성 분석
상추의 생중량은 농촌진흥청 표준분석법에 준하여 구중으로 측정하였다 (RDA, 2012). 수확한 상추는 60°C에서 7일간 건조한 후 건물중 (dry weight, DW)을 측정하였으며, 이후 분쇄 및 균질화하여 질소 (N), 인 (P), 칼륨 (K), 칼슘 (Ca) 및 마그네슘 (Mg) 흡수량을 분석하였다. 질소는 Kjeldahl법에 따라, P, K, Ca 및 Mg은 HNO3 습식분해 (wet digestion) 후 ICP-OES (BRE731400, Thermo Scientific, USA)로 정량하였다. 상추 양분 흡수량은 각 양분 함량과 건물중의 곱으로 산출하였다.
통계분석
본 연구에서는 목재 바이오차 처리수준에 따른 토양 및 식물체 특성 차이를 비교하기 위해 일원분산분석 (one-way ANOVA)과 사후분석 (Turkey’s HSD)을 수행하였다. 또한 바이오차 처리수준 (application rate, R), 재배시기 (season, S) 및 이들 교호작용 (R x S)이 각 특성 변화에 미치는 영향을 알아보기 위해 이원분산분석 (Two-way ANOVA)을 수행하였다. Pearson correlation 분석을 통해 변수간 상관관계를 알아보았으며, 모든 통계분석은 Minitab16 (Minitab Inc., USA)을 이용하여 수행하였다.
Results and Discussion
목재 바이오차 시용에 따른 토양 화학성 변화
바이오차 처리에 따른 상추재배 토양의 화학적 특성 변화는 Table 3과 같다. 토양 pH는 1차 재배 후 바이오차 처리수준이 증가할수록 점차 증가하는 경향 (pH 6.7 - 7.0)을 나타내었으나, 대조구 토양 (pH 6.6)과는 유의한 차이가 없었다 (p > 0.05). 반면 2차 재배 후에는 모든 바이오차 처리구의 토양 pH (7.3 - 7.4)가 대조구 (pH 7.1)보다 유의하게 높았다 (p < 0.05). 또한 이원분산분석 결과를 미루어 볼 때, 바이오차 처리수준보다는 연용 시비가 토양 pH 변화에 큰 영향을 미치는 것으로 보였다 (p < 0.001). 이와 같이, 바이오차 처리에 따른 토양 pH 증가는 다양한 작용기 (e.g., hydroxyl-, carbonyl-, carboxyl group, etc.), 탄산염, 수산화물, 황산염 등으로 구성된 바이오차의 높은 pH 특성으로부터 기인된 시용 효과로 보인다 (Yuan et al., 2011; Cheah et al., 2014; Fidel et al., 2017). 따라서, 상추 재배 시 토양 적정 pH가 6.5 - 7.0임을 감안할 때, 지속적인 바이오차 연용 시비는 토양의 알카리화를 유발하여 작물의 생육장해를 초래할 수 있을 것으로 판단된다. 따라서, 바이오차 연용 시 증가되는 토양 pH를 개선하기 위해서 인산석고 (phosphogypsum, CaSO4 ‧ 2H2O), 유안 ((NH4)2SO4), 황산칼륨 (K2SO4) 등과 같은 첨가제 혼용이 필요할 것으로 판단된다.
토양 내 유기물 함량은 바이오차 시용량과 양의 상관관계를 보였다: 1차 재배 (r = 0.882, p < 0.001) 및 2차 재배 (r = 0.949, p < 0.001). 하지만 바이오차 처리구 중 WB400 (1차: 19.5 g kg-1, 2차: 22.4 g kg-1)과 WB800 (1차: 19.9 g kg-1, 2차: 24.9 g kg-1)는 대조구 (1차: 16.2 g kg-1, 2차: 18.9 g kg-1)보다 유의하게 높은 유기물 함량이 관찰되었다 (p < 0.05). 게다가, 이원분산분석 결과에 따르면 토양 중 유기물 함량 변화는 바이오차 시용량 (p < 0.01), 시용횟수 (p < 0.001) 뿐만 아니라 이들의 교호작용 (p < 0.05)에 의한 영향이 모두 큰 것으로 나타났다. 이와 같이, 난분해성 탄소화합물로 구성된 바이오차는 시용량 증가 및 연용 시비로 인해 토양 내 누적효과가 큼으로, 토양 중 탄소 격리 효과에 탁월한 것으로 사료된다 (Ding et al., 2023).
토양 중 유효인산 함량 또한 바이오차 처리수준이 증가함에 따라 증가하는 경향을 보였다: 1차 재배 (r = 0.658, p < 0.01) 및 2차 재배 (r = 0.816, p < 0.001). 1차 재배 후 토양 중 유효인산 함량은 대조구 (64.8 mg kg-1)에 비해 WB400 및 WB800 처리구 (74.3 mg kg-1)에서 14 - 15% 높았으며 (p < 0.05), 2차 재배 후에는 WB800 처리구 (79.3 mg kg-1)에서 대조구 (61.4 mg kg-1)보다 약 29% 높았다 (p < 0.05). Wang et al. (2021)의 연구에 따르면, 볏짚 바이오차 15 Mg ha-1를 토양에 2년 연용 시비하였을 때 대조구에 비해 토양 내 유효인산 함량이 약 2.7배 증가하였다. 이러한 결과는 바이오차의 작용기들이 철 및 알루미늄 산화물과 같은 토양 미네랄에 의한 인의 흡착을 감소시키거나 (Morales et al., 2013), 바이오차에서 용출되는 용해성 유기물질 (dissolved organic matter substances)이 활성 알루미늄 및 철과 킬레이트화되어 인의 유효도를 증가시킨 것으로 사료된다 (Schneider and Haderlein, 2016). 그러나, 이원분산분석 결과에 따르면 토양 내 유효인산 함량은 바이오차의 시용 횟수에는 유의한 영향을 받지 않는 것으로 나타났다 (p = 0.951, Table 3). 이 외에, 양이온교환용량 (CEC) 및 교환성양이온 (Exch. K, Ca) 함량은 바이오차 처리량에 따라 유의한 차이가 없었다.
Table 3.
Chemical properties of soils treated with different application rates of wood-derived biochar (WB) after two cultivations of lettuce.
Season | Treatment |
pH (1:5w) |
EC (dS m-1) |
OM (g kg-1) |
T-N (%) |
Av. P2O5 (mg kg-1) |
CEC (cmolc kg-1) |
Exch. K (cmolc kg-1) |
Exch. Ca (cmolc kg-1) |
Exch. Mg (cmolc kg-1) |
1st cultivation | Control | 6.6 a1 | 0.59 a | 16.2 b | 0.11 a | 64.8 b | 13.7 a | 0.7 a | 9.5 a | 3.4 a |
WB100 | 6.7 a | 0.67 a | 16.8 b | 0.11 a | 68.0 ab | 14.0 a | 0.8 a | 9.8 a | 3.5 a | |
WB200 | 6.8 a | 0.53 a | 18.0 ab | 0.11 a | 73.5 ab | 14.3 a | 0.7 a | 9.3 a | 3.2 a | |
WB400 | 6.9 a | 0.46 a | 19.5 a | 0.11 a | 73.9 a | 13.6 a | 0.7 a | 9.4 a | 3.3 a | |
WB800 | 7.0 a | 0.42 a | 19.9 a | 0.10 a | 74.3 a | 14.1 a | 0.7 a | 9.5 a | 3.3 a | |
2nd cultivation | Control | 7.1 b | 0.31 a | 18.9 c | 0.11 a | 61.4 b | 13.7 a | 0.9 a | 9.8 a | 3.5 a |
WB100 | 7.4 a | 0.21 a | 20.4 c | 0.11 a | 69.6 ab | 13.9 a | 1.0 a | 10.2 a | 3.6 a | |
WB200 | 7.3 a | 0.23 a | 20.0 c | 0.10 a | 67.8 ab | 13.9 a | 0.9 a | 10.0 a | 3.4 a | |
WB400 | 7.3 a | 0.25 a | 22.4 b | 0.12 a | 72.6 ab | 13.7 a | 0.9 a | 10.3 a | 3.5 a | |
WB800 | 7.4 a | 0.18 a | 24.9 a | 0.11a | 79.3a | 14.1 a | 1.0 a | 10.0 a | 3.4 a | |
Two-way ANOVA (p-value) | Application rate (R) | 0.4982 | 0.073 | 0.002 | 0.054 | <0.001 | 0.224 | 0.065 | 0.001 | 0.005 |
Season (S) | <0.001 | <0.001 | <0.001 | 0.833 | 0.951 | <0.001 | <0.001 | 0.012 | 0.290 | |
Interaction (R x S) | 0.027 | 0.247 | 0.011 | 0.119 | 0.472 | 0.503 | 0.415 | 0.030 | 0.388 |
목재 바이오차 시용에 따른 상추 생산성 변화
바이오차의 처리 수준 및 시용 횟수는 상추 생중량에 유의한 영향을 미쳤으며 (p < 0.001), 바이오차 처리 수준별 상추 생중량은 Fig. 1와 같다. 1차 재배 시 상추의 생중량은 국내 바이오차 적정 시용량의 50%를 처리한 WB100 처리구 (99.0 g pot-1)에서 가장 높았으며, 그 다음 WB200 (92.4 g pot-1), WB400 (89.2 g pot-1), control (79.9 g pot-1) 및 WB800 (72.0 g pot-1) 순으로 높았다(Fig. 1A, p < 0.05). 특히, 다른 바이오차 처리구보다 WB800 처리구의 상추 생중량이 유의하게 낮았는데 (p < 0.05), 이는 과도한 바이오차 시용이 작물생산성에 악영향을 미칠 수 있음을 시사한다. 이와 유사하게 Akumuntu et al. (2024)의 연구에 따르면 상추의 가식부 (shoot) 생중량이 고농도 (>1%)의 바이오차 시용에 의해 대조구보다 낮았다. 이는 과량의 바이오차 처리로 인한 양분 흡착 (Sorensen and Lamb, 2016) 및 용적밀도 증가에 따른 배수 불량 (Humberto, 2017) 등에 의한 것으로 사료된다.
목재 바이오차를 연용 시비 후 상추를 2차 재배한 결과, 상추의 생중량은 WB200 처리구 (50.6 g pot-1)에서 가장 높았으며 다음으로 WB100 (40.0 g plot-1), WB400 (37.6 g pot-1), control (30.6 g pot-1) 및 WB800 (24.6 g pot-1) 순으로 높았다 (Fig. 1B, p < 0.05). 본 연구결과와 유사하게도 Yi et al. (2019)은 2 Mg ha-1의 목재 바이오차 처리가 상추의 수확량을 최대화하는데 가장 적절한 시용수준인 것을 산출하였다. 또한, Kim et al. (2022b)의 연구에서도 2 Mg ha-1의 왕겨 바이오차 처리를 통해 가장 높은 수박의 수량 증수효과가 나타났다. 반면 1차 재배 시 동일한 결과가 유출되지 않았는데, 이는 2차 재배와 달리 토양 내 처리된 바이오차가 충분한 기간 (20일 이상)동안 안정화되지 않은 상태에서 재배시험을 수행하여 비롯된 것으로 사료된다 (Kang et al., 2017).
게다가 전반적으로 2차 재배 시 상추 생중량이 1차 재배 시보다 적은 것은 비록 두 재배시험이 동일한 기간 동안 수행되었지만 재배시기별로 평균온도, 일조량, 일교차 등 환경요인의 차이로 인해 작물의 생장속도에 영향을 미쳤기 때문으로 보인다. 그럼에도 불구하고 목재 바이오차 시용에 따른 상추의 최대 수확량을 다항 회귀분석으로 산출한 결과, 1차 및 2차 수확 시 각각 3.04 Mg ha-1 및 3.36 Mg ha-1로 유사하게 나타났다.
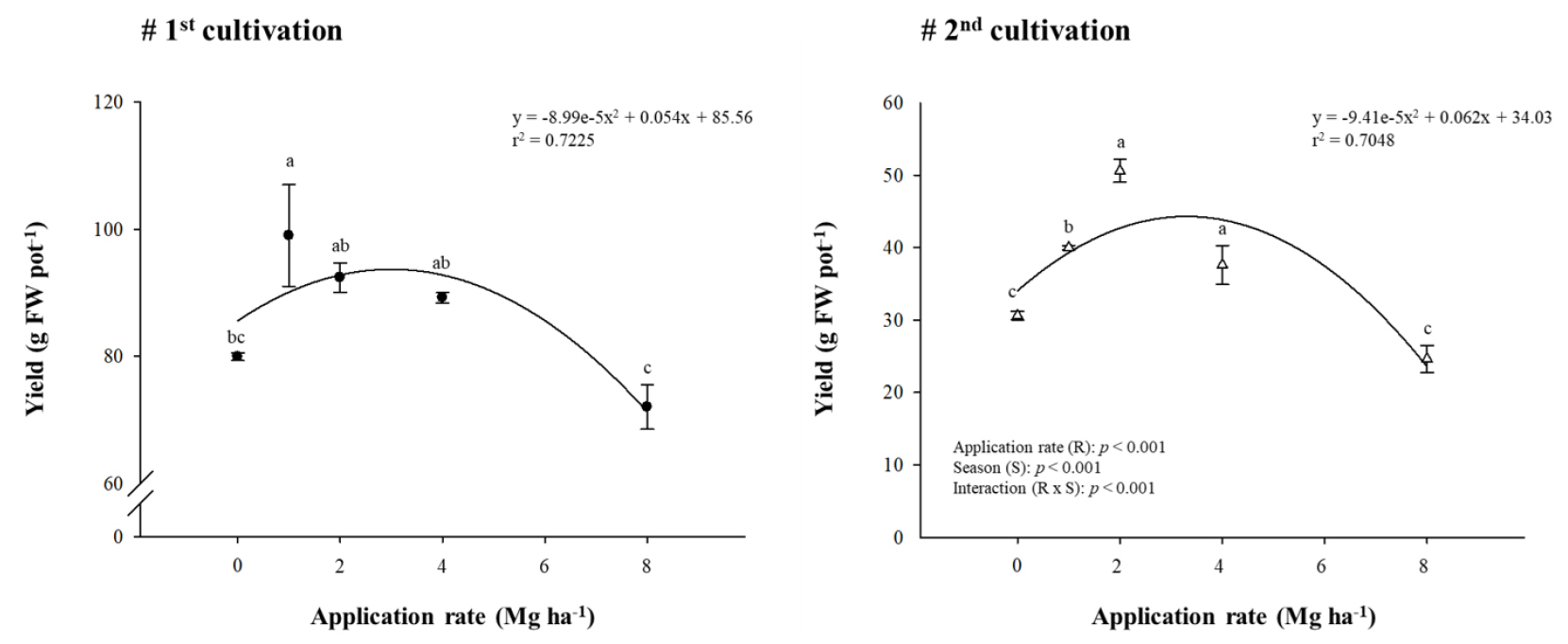
Fig. 1.
Polynomial relationships between fresh weights of lettuce and application rates of wood-derived biochar at the first (A) and second (B) cultivation. Data indicate the mean of three replicates (n = 3). Different letters represent significant differences among the treatments (Tukey’s HSD, p < 0.05). Two-way ANOVA analysis shows the effect of biochar application rates (R), season (S), and their interaction (R x S).
목재 바이오차 시용에 따른 상추 양분 흡수량 변화
목재 바이오차 처리수준에 따른 재배시기별 상추의 양분 흡수량 변화는 Table 4와 같다. 1차 재배 후 상추 가식부 중 양분 흡수량을 처리구별로 비교하였을 때, WB100 처리구에서 모든 양분 흡수량이 가장 높게 나타났다 (p < 0.05). 또한, 질소와 칼륨을 제외한 나머지 양분들은 바이오차 처리량이 증가함에 따라 감소하는 경향을 보였다. 이와 달리, 2차 재배 후 상추의 모든 양분 흡수량은 WB200 처리구에서 가장 높았으며, 이보다 바이오차 처리량이 높을수록 양분 흡수량은 감소하였다. 특히, 바이오차 과량 처리구인 WB800에서는 마그네슘 흡수량이 두 재배 시기 모두 대조구보다 유의하게 낮았다 (p < 0.05). 따라서, 이들을 종합하여 진행한 이원분산분석 결과, 작물의 양분 흡수량은 바이오차의 처리 수준 및 시용 횟수에 유의적인 영향을 받는 것으로 판단된다 (p < 0.001).
Table 4.
Dry weight (DW) and nutrient absorption content in lettuce grown under different application rates of wood-derived biochar (WB) at two cultivations.
Season | Treatment |
DW (g) |
N (mg plant-1) |
P (mg plant-1) |
K (mg plant-1) |
Ca (mg plant-1) |
Mg (mg plant-1) |
1st cultivation | Control | 6.02 | 122.2 b1 | 8.6 c | 128.5 ab | 61.5 b | 7.7 ab |
WB100 | 7.22 | 167.7 a | 11.7 a | 149.1 a | 74.3 a | 8.8 a | |
WB200 | 7.11 | 139.4 b | 10.5 ab | 143.6 a | 62.8 ab | 7.7 ab | |
WB400 | 7.29 | 148.5 ab | 10.2 abc | 149.7 a | 62.3 ab | 6.6 bc | |
WB800 | 5.98 | 127.1 b | 8.9 bc | 116.7 b | 58.7 b | 5.8 c | |
2nd cultivation | Control | 2.42 | 25.6 cd | 5.9 cd | 72.0 b | 19.0 c | 6.5 ab |
WB100 | 2.72 | 29.5 bc | 6.6 bc | 90.1 a | 23.6 b | 6.0 b | |
WB200 | 3.19 | 43.9 a | 7.7 a | 95.8 a | 30.7 a | 7.2 a | |
WB400 | 3.08 | 30.9 b | 6.9 ab | 91.2 a | 26.9 ab | 6.1 b | |
WB800 | 2.39 | 22.6 d | 5.5 d | 62.8 b | 23.4 b | 4.7 c | |
Two-way ANOVA (p-value) | Application rate (R) | 0.2772 | <0.001 | <0.001 | <0.001 | <0.001 | <0.001 |
Season (S) | <0.001 | <0.001 | <0.001 | <0.001 | <0.001 | <0.001 | |
Interaction (R x S) | 0.863 | <0.001 | <0.001 | 0.059 | <0.001 | <0.001 |
Conclusions
본 연구는 목재 바이오차의 연용 처리가 밭 토양의 화학적 특성과 상추 수량 및 품질에 미치는 영향에 대해 알아보고자 수행되었다. 목재 바이오차의 연용 시비는 토양 pH와 유기물 및 유효인산 함량에 큰 영향을 미쳤다. 가장 높은 시용량 처리구 (WB800)에 의해 2차 재배 후 토양 pH는 7.1에서 7.4까지 증가하였으며, 유효인산 및 유기물 함량 또한 대조구 대비 각각 29% 및 32% 증가하였다. 반면 연작 재배 후 WB200 처리구에서 상추의 양분 흡수량 (N, P, K, Ca, Mg)이 가장 높았으며, 수확량은 대조구 대비 65% 증수효과가 확인되었다. 하지만, 목재 바이오차를 과량 처리 (i.e., WB800)할 시 토양 알칼리화, 배수 불량 등이 야기되어 작물의 생육이 저해되는 것으로 보였다. 다항 회기분석을 통해 상추 연작 재배 시 바이오차 적정 시용량을 산출한 결과, 3.36 Mg ha-1가 나타났다. 하지만 본 연구에서는 제한된 환경과 단기간 연용 시비 효과만을 알아보았기에, 이 결과를 직접 현장에 적용하는데 어려움이 따를 것으로 예상된다. 따라서, 현장에서 목재 바이오차 활용 효율성을 높이기 위해서는 바이오차 연용 시비효과에 대한 장기적인 연구가 필요하다고 판단된다.