Introduction
Materials and Methods
Preparation of bacterial strain and fungal pathogen
Antagonistic activity of B. velezensis CE 100 against D. citri
Growth pattern of B. velezensis CE 100 and pH changes in large-scale culture
Growth pattern of B. velezensis CE 100 and pH changes in small-scale culture
Inhibition on mycelial growth of D. citri by bacterial culture filtrate (BCF)
Effect of bacterial culture filtrate on conidial germination
Qualitative enzyme assay
In vivo evaluation of melanose control by strain CE 100
Statistical analysis
Results
Antifungal activity of B. velezensis CE 100 against D. citri
Growth pattern of B. velezensis CE 100 and pH changes in small scale and large-scale cultivation
Mycelial growth inhibition of D. citri by BCF of strain CE 100
Inhibition of D. citri conidial germination by BCF of strain CE 100
Qualitative enzymatic activity of BCF from large-scale cultivation
In vivo biocontrol efficacy of B. velezensis CE 100 against D. citri
Discussion
Conclusions
Introduction
Citrus fruits are an excellent source of essential nutrients, necessary for body health. It could offer other health benefits, including antioxidants and antimutagenic properties that reduce the risk of various diseases, such as hypertension, cancer, asthma and cardiovascular and heart diseases (Turner and Burri, 2013). Jeju island in South Korea is rich in citrus resources, and mandarins (Citrus reticulata Blanco) are the largest produced fruit among the citrus fruits. Since the 1970s, the citrus industry has grown dramatically, and about 600,000 tonnes are produced from 25,000 hectares, contributing more than 40% of Jeju’s agricultural income (Kwon et al., 2003; Hyun et al., 2011). Fungal diseases such as melanose, caused by Diaporthe citri; scab, caused by Elsinoe fawcettii; and postbloom fruit drop, caused by Collectotrichum acutatum, pose a great challenge to citrus production worldwide (Timmer et al., 2004). Among these, melanose causal agents, D. citri F.A. Wolf. (sexual phase) and Phomopsis citri H. Fawc (asexual phase) cause small black lesions surrounded by a yellow zone on leaves, and severe infection causes distorted leaves. Melanose produces external blemishes on the fruits, resulting in loss of fresh fruit value and a decrease in marketability (Gopal et al., 2014).
Most of the control strategies against melanose depend on chemical fungicides, which decrease the severity of the disease in host plants. However, repeated applications of chemical fungicides cause toxic effects on the environment and lead to an emergence of new resistant pathogens (Aktar et al., 2009). Even though copper-based fungicides play an important role in plant protection, they could have adverse effects on food safety, besides causing phytotoxic effects to plants (Hyun et al., 2005). Therefore, biological control (using microbial antagonists) is a safer alternative to chemical fungicides, contributing to an eco-friendly agriculture, maintaining high quality and safety of fruits, and improving agricultural income (Droby, 2006). Various kinds of biocontrol agents have been developed for field applications (Bejarano and Puopolo, 2020). Among several biocontrol agents, species within genus Bacillus are known to be effective agents against plant pathogens through secretion of a wide spectrum of antimicrobial substances and lytic enzymes. Moreover, their resistance to extreme environmental conditions including temperature, pH, and drought by forming endospores makes them more attractive to be used in plant disease control (Shafi et al., 2017).
Several reports have revealed the potential of various biocontrol agents. However, there are limited studies about their performance under field conditions. Although active substances including enzymes and antibiotics can be secreted by biocontrol agents during mass production under controlled conditions, it is hard to release high concentration of such metabolites under field conditions (Köhl et al., 2019). Therefore, application of large quantities of cells and active ingredients through large-scale cultivation in cost-effective medium could be considered as a solution to reduce constraints of low population of biocontrol agents and hence low efficacy. As mass production in liquid culture is the most cost-effective method to produce most microbial agents (Jackson, 1997), the large-scale cultivation of biocontrol agents in cheap liquid medium and the delivery of this fresh mixture of biocontrol agents and active substances to the field could be a reliable approach to successful plant disease control. However, several parameters including pH, temperature and agitation are important factors for successful cultivation. The pH is an important culture condition during microbial growth that is related to growth and survival. In addition, pH also influences the activities of extracellular enzymes and desired metabolite production (Slininger and Shea-Wilbur, 1995; Jin and Kirk, 2018). In our previous studies, the inhibitory effect of the soil originated Bacillus velezensis CE 100 has been reported on growth of different fungal pathogens and root knot nematodes via the action of antifungal compounds and lytic enzymes (Choi et al., 2020; Choub et al., 2021; Moon et al., 2021). Therefore, the objectives of our study were to investigate large-scale cultivation of B. velezensis CE 100 in cost-effective medium using low-cost fermenter and to determine the biocontrol efficacy of B. velezensis CE 100 against citrus melanose caused by D. citri.
Materials and Methods
Preparation of bacterial strain and fungal pathogen
Bacterial strain used in this study was Bacillus velezensis CE 100, which was isolated from the soil of tomato plant (accession number: KACC 81101BP, Korean Agricultural Culture Collection). The strain was streaked on Tryptone soy agar (TSA; tryptone 17 g L-1, soy peptone 3 g L-1, NaCl 5 g L-1, K2HPO4 2.5 g L-1, dextrose 2.5 g L-1, agar 20 g L-1) medium to obtain the pure colonies, which were inoculated again in Tryptone soy broth (TSB) medium for three days at 30°C and 130 rpm on a shaking incubator. The resulting bacterial broth was adjusted to 107 CFU mL-1 and maintained with 50% (v/v) glycerol solution at -80°C for further experiment.
The fungal pathogen Diaporthe citri F.A. Wolf was obtained from Korean Agricultural Culture Collection (KACC) with accession number KACC 45782 and sub-cultured on potato dextrose agar (PDA; potato starch 4 g L-1, dextrose 20 g L-1, agar 20 g L-1) medium at 26°C for 14 days. The conidial suspension of D. citri for in vitro and in vivo assays was prepared by the method described previously by Ko et al. (2012). A 5-mm-diameter mycelial plug which was taken from a freshly growing culture using a sterile cork borer, was grown on PDA medium for 14 days at 26°C. To induce conidial production, the fresh fungal plug was inoculated again on oatmeal agar (OA) medium for 14 days at 26°C, then were grown at room temperature under fluorescent light for 14 days. Then the fully sporulated plate was flooded with 10 mL of sterile distilled water and the mycelium were scratched using a sterile spatula. The collected conidia were filtered through sterile miracloth (CALBIOCHEM) and the number of conidia was counted with the aid of a hemocytometer under a microscope (Olympus BX41, Japan) at 300× magnification and adjusted to 1.0 × 106 conidia mL-1.
Antagonistic activity of B. velezensis CE 100 against D. citri
The antagonistic activity of B. velezensis CE 100 against D. citri was assessed by the dual-culture method. The strain CE 100 was streaked on one side of PDA plate and the 2-mm freshly grown D. citri fungal plug was placed on the other side of the inoculated plates. The plate inoculated with D. citri alone was used as a control. The plates were incubated at 25°C for 14 days and growth inhibition of D. citri was calculated using the formula:
where, R1 : The radial growth of D. citri in the control plate
R2 : The radial growth of D. citri in the dual culture plate
Growth pattern of B. velezensis CE 100 and pH changes in large-scale culture
According to the report by Lee et al. (2019), the low-cost, Pink-Brown (PB) medium was used for mass production of strain CE 100. The composition of PB medium (per litre) was compound fertilizer 3.0 g, MgSO4 0.2 g, KH2PO4 0.2 g, NaCl 0.1 g, sucrose 3.0 g, chitin powder 0.5 g, yeast extract 0.6 g. The experiment was conducted using M-1000 fermenter (MC-Biotech, South Korea) containing 500 L non-sterilized PB medium (Lee et al., 2019). Three independent experiments were done to observe the growth pattern of strain CE 100 and pH changes during the incubation days. First, 100 µL (107 CFU mL-1) of strain CE 100 was spread on 1% TSA plates (150 mm diameter) using sterile glass beads and incubated for three days at 30°C. Two plates of bacterial strain were inoculated in a large-scale fermenter containing 500 L of non-sterilized PB medium, and the temperature was kept at 50°C to inhibit the growth of other mesophilic bacteria. The samples from the fermenter were taken daily to investigate the cell numbers and changes of pH for 10 days. This study was carried out to confirm the growth of strain CE 100 in a large-scale fermenter under thermophilic condition as compared to the growth of this strain in lab-scale cultivation.
Growth pattern of B. velezensis CE 100 and pH changes in small-scale culture
To study the growth pattern of B. velezensis CE 100 and changes in pH during the incubation days, TSB medium and PB medium were used for small-scale cultivation (in lab) of B. velezensis CE 100. The 10 µL of strain CE 100 broth culture (107 CFU mL-1) was dropped on TSA medium and incubated at 30°C to attain identical colonies, which were used for small-scale inoculation of strain CE 100. Then 500 mL of TSB and PB medium were prepared, and the initial pH was adjusted to 7.5 with KOH. Each medium was sterilized by autoclaving. Strain CE 100 was inoculated in each medium and incubated at 50°C. The samples were collected from each broth culture every day for 10 days to examine changes of pH and the cell numbers of each sample from each sampling day were observed using plate count method.
Inhibition on mycelial growth of D. citri by bacterial culture filtrate (BCF)
To investigate the inhibitory effect of BCF on mycelial growth of D. citri, the 5, 7, and 10 days old bacterial culture broths from small-scale and large-scale cultivation were centrifuged at 12,000 rpm for 15 min, and the supernatants were filtered through Whatman No. 6 filter paper. The remaining live cells and endospores were eliminated completely using a syringe filter with a 0.2 µm pore size. The resulting bacterial culture filtrate (BCF) was mixed with the sterilized and cooled PDA medium, to prepare the final concentrations of 10%, 30%, and 50%. Then a D. citri mycelial plug (3-mm-diameter) was inoculated in the centre of each plate. D. citri grown on PDA plate without BCF (0%) was used as a control. Mycelial growth inhibition (%) was measured at 14 days after inoculation and the inhibition percent was calculated using the equation,
where, D : Fungal-colony diameter in the control plate
d : Fungal-colony diameter in the BCF-treatment plate
Then, a small piece of mycelium from each plate was used to examine the hyphal deformation of D. citri affected by BCF under a light microscope (Olympus BX41, Japan).
Effect of bacterial culture filtrate on conidial germination
To examine the inhibition on conidial germination, the BCF from the large-scale culture was mixed with the autoclaved PDB broth to prepare final concentrations of 10%, 30%, 50%, and 70%. Then 100 µL of conidial suspension (1.0 x 106 conidia mL-1) was inoculated into each tube to make a total volume of 1 mL. Only the conidial suspension inoculated in PDB broth without BCF (0%) was used as a control. The experiment was conducted with three replicates. The number of germinated conidia was counted with the aid of the hemocytometer, and the germination inhibition percent was calculated as based on the control.
Qualitative enzyme assay
The media for different enzyme activities were prepared by mixing 2% agar with different substrates, such as 2% casein for protease activity, 2% starch for amylase activity, 1% laminarin for glucanase activity, and 1% colloidal chitin for chitinase activity. A cellulase medium was prepared using cellulose 0.5 g, Congo red 0.1 g, and agar 20 g per litre. The medium consisting of phenol red 0.1 g, agar 20 g, and olive oil 20 mL per litre (pH 7.3) was used to detect lipase activity. Then an 8-mm well was made in each agar medium, and 100 µL of BCF prepared from a seven-days-old broth culture from large-scale cultivation of strain CE 100 was inoculated into the well and incubated at 30°C for 1 day. After that, the plates were flooded with iodine solution (iodine 1 g, potassium iodide 5 g, and distilled water 330 mL). The development of a clear zone around the well indicated the production of the respective enzyme.
In vivo evaluation of melanose control by strain CE 100
Three-year-old mandarin seedlings were purchased from Jeju Island, South Korea, and transplanted into identical plastic pots (30 cm × 25 cm) containing 2:1:1 ratio of bed soil, vermiculite, and granite fragments. The pots were kept in a glasshouse at Chonnam National University for a year with routine irrigation. Then, the mandarin plants were used to investigate melanose disease control by the broth culture of strain CE 100. All leaves and small branches of each plant were cut and the plants were grown again. The plants with new young leaves were used for three different treatments including treatment, with strain CE 100 broth culture (CE 100), a synthetic fungicide (F), and water as control (Con) in this experiment and the plants were arranged by a completely randomized design with three replications. Strain CE 100 was grown in PB medium using a large-scale fermenter for seven days at 50°C. Diluted strain CE 100 culture broth was prepared by mixing culture broth (2.0 × 105 CFU mL-1) with distilled water (1:1, v/v). Then the diluted culture was sprayed on the leaves of the plant and air-dried for 2 h. According to the instructions of the product, the commercial synthetic fungicide (Dithianon) was prepared and used to spray on the leaves for fungicide treatment and air-dried for 2 h. For control, water was used to spray on the leaves of the plants. Then, the plants of each treatment were inoculated with the conidial suspension of D. citri (1.0 x 105 conidia mL-1) through foliar spraying. The plants were then kept at 28 ± 2°C and 60% RH. The development of melanose symptoms was examined every day and the disease incidence was assessed at 20 days after inoculation with D. citri by counting the number of leaves having brown lesions with yellow margins on the plants of each treatment.
Statistical analysis
Statistical analysis of the experimental data was performed by analysis of variance (ANOVA) using the statistical analytical system (SAS. Version 9.4). The mean comparison was done by the least significant difference (LSD) tests at p < 0.05.
Results
Antifungal activity of B. velezensis CE 100 against D. citri
The dual-culture assay showed that strain CE 100 strongly inhibited growth of the target fungal pathogen D. citri with 73.1% at 10 days after inoculation (Fig. 1).
Growth pattern of B. velezensis CE 100 and pH changes in small scale and large-scale cultivation
In the lab-scale experiment, growth of strain CE 100 in TSB medium was stable at around 106 CFU mL-1 during the experimental period, and the maximum growth was 5.77 × 106 CFU mL-1 at 2 days after inoculation (DAI), whereas the highest growth rate of strain CE 100 in PB medium (2.13 × 106 CFU mL-1) was found at 2 DAI, slowly decreased, and reached 0.5 × 106 CFU mL-1 at the end of the experiment (Fig. 2a). However, a similar trend of pH changes was observed for both tested media in small-scale inoculation of strain CE 100, which showed a sudden decline (6.21 and 5.74) at 1 DAI and gradual increase starting from 2 DAI until 10 DAI; the final pH values of the TSB and PB media were 7.54 and 7.2 (Fig. 2b). On the other hand, for the 500 L large-scale inoculation, the growth rate of strain CE 100 was the highest at 3 DAI (2.67 × 105 CFU mL-1), and the growth rate did not change much during the experimental period (Fig. 2a). The relative pH of the medium rapidly dropped to the lowest value (4.75) at 1 DAI and then steadily increased from 2 DAI to the end of the experiment, when the value reached 5.86 (Fig. 2b).
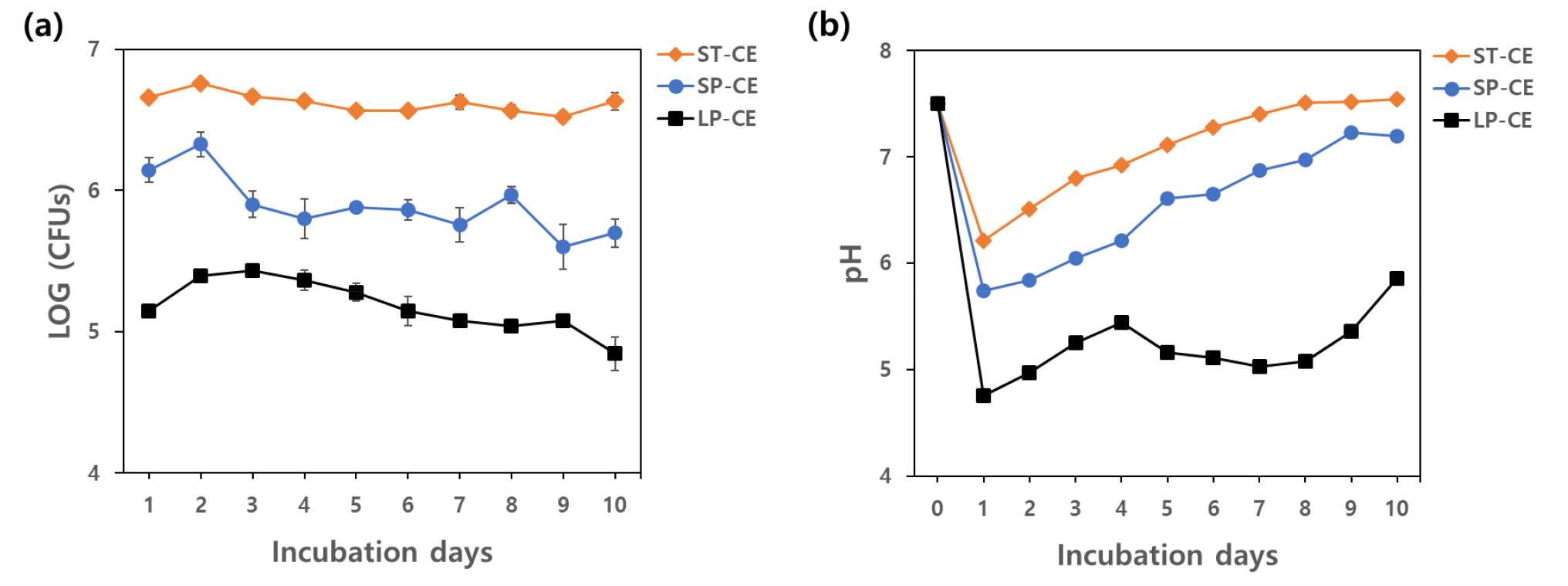
Fig. 2.
Growth of B. velezensis CE 100 (a) and changes of pH (b) during small-scale inoculation in two different media and large-scale inoculation in PB medium at 50°C for 10 days (ST-CE: small-scale inoculation of strain CE 100 in TSB medium; SP-CE: small-scale inoculation of strain CE 100 in PB medium; LP-CE: large-scale inoculation of strain CE 100 in PB medium).
Mycelial growth inhibition of D. citri by BCF of strain CE 100
Mycelial growth of D. citri was differentially inhibited by different BCF concentrations of B. velezensis CE 100 from both small- and large-scale cultivation using TSB and PB media. The inhibition level increased with the increased concentration of BCF prepared from a large scale in PB medium (LP-CE) and a small scale in TSB medium (ST-CE). The most significant effect was observed for the treatment with 50% BCF from LP-CE of 5- and 7-day cultures (59.27 and 63.31%) and from ST-CE of a 7-day culture (63.31%). However, the degree of inhibition was inversely related to the concentration of BCF from small-scale cultivation in the PB medium (SP-CE). The lower BCF, the higher the inhibition on the fungal pathogen (Fig. 3). The SP-CE had weaker inhibition of the target fungal pathogen than did LP-CE at every BCF concentration. Moreover, the microscopic examination clearly revealed that hyphal morphology of the pathogen was seriously affected by BCF of the strain CE 100, showing the abnormal growth with swelling and twisting along the hyphal branch as compared to the straight and normal hyphal structure of the control (Fig. 4).
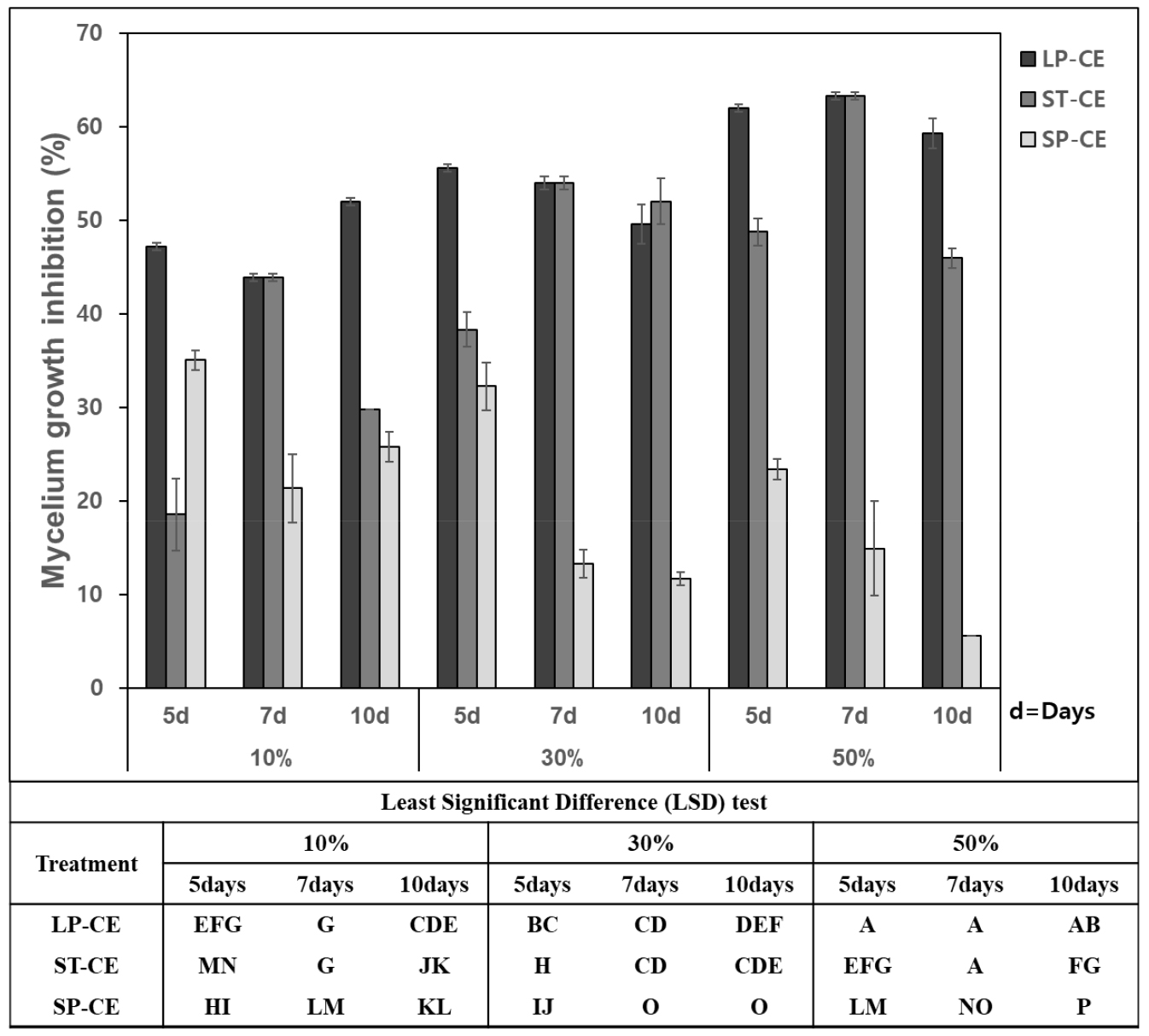
Fig. 3.
Mycelial growth inhibition of D. citri by different BCF concentrations of the strain CE 100 from the large-scale cultivation in PB medium (LP-CE), the small-scale cultivation in TSB medium (ST-CE) and PB medium (SP-CE) of different (5, 7 and 10) inoculation days. The values represent the means calculated from three replicates and means with the same letter are not significantly different at p < 0.05 by least significant difference test.
Inhibition of D. citri conidial germination by BCF of strain CE 100
The results in Table 1 show that BCF of B. velezensis CE 100 had a strong inhibitory effect on the germination of D. citri conidia. The concentration of BCF influenced the intensity of the inhibition of the germination. An inhibition rate of more than 96% was observed using the highest concentration (70% BCF). The presence of even lower concentrations of BCF (10%) caused D. citri conidia germination to be slightly limited, whereas 100% of conidia germinated in the control treatment after 24 h.
Table 1
Inhibition on conidial germination of D. citri by BCF from large-scale culture of B. velezensis CE 100 at 25 °C for 24 h. The values represent the means calculated from three replicates and means with the same letter are not significantly different at p < 0.05 by least significant difference test.
Culture filtrate of B. velezensis CE | 10% | 30% | 50% | 70% |
Inhibition of spore germination (%) | 9.27 d | 29.85 c | 86.71 b | 98.3 a |
Qualitative enzymatic activity of BCF from large-scale cultivation
Qualitative enzymatic activities of the BCF from large-scale cultivation of strain CE 100 were measured, as presented in Table 2, which clearly shows that the BCF of strain CE 100 had five different enzyme activities involving glucanase, protease, chitinase, lipase, and cellulase by developing the clear zone around the well except for amylase enzyme.
Table 2
Qualitative enzyme activity of BCF from large scale culture of B. velezensis CE 100.
Enzyme | Activity |
Amylase | - |
Cellulase | + |
Chitinase | + |
Glucanase | + |
Protease | + |
Lipase | + |
In vivo biocontrol efficacy of B. velezensis CE 100 against D. citri
The foliar spray with B. velezensis CE 100 culture on mandarin plants had a remarkable effect on control of melanose on leaves. The lowest disease incidence was observed in the plants treated with the commercial fungicide with 3.51% (Fig. 5). Treatment with the biocontrol strain CE 100 significantly reduced melanose disease incidence (9.32%) compared to the control treatment, which had the highest disease incidence (46.28%) at 20 days after pathogen inoculation. There was no significant difference between treatments with strain CE 100 and the commercial fungicide.
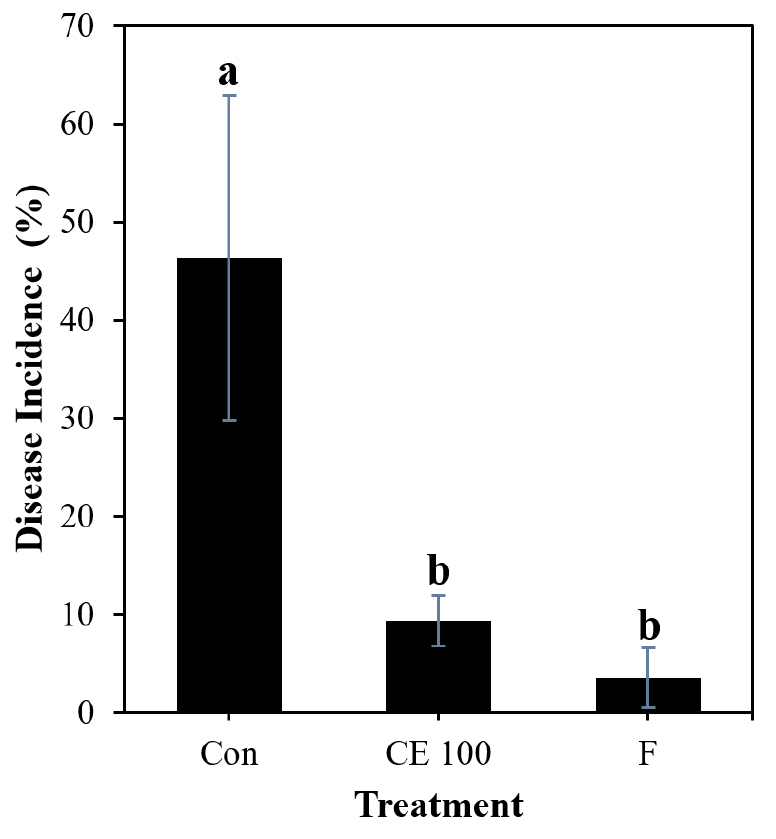
Fig. 5.
Effect of different treatments on melanose disease incidence on leaves of mandarin plants at 20 days after inoculation with D. citri. Statistical comparison between treatments was done by least significant difference (LSD) test at p < 0.05 level. Means with the same letter are not significantly different from each other. *Con- Control, CE 100- Treatment with B. velezensis CE 100 culture, F- Treatment with fungicide
Discussion
Bacillus strains are well-known for antibiotic production and release diverse antimicrobial compounds, such as iturins, surfactin, fengycin which are responsible for their inhibitory effect against a wide range of phytopathogens (Meena and Kanwar, 2015). In other studies, the growth of Glomerella cingulata was restricted by B. velezensis AR1 (Regassa et al., 2018), and the strong antagonistic effect of B. velezensis S3-1 on the growth of Botrytis cinerea has been recently reported by Jin et al. (2017). Furthermore, the pre-inoculation of ten antagonistic Bacillus strains reduced more than 50% of green mold caused by Penicillium digitatum on the surface area of oranges under in vitro conditions (Abraham et al., 2010). In this study, the antifungal effect of B. velezensis CE 100 against D. citri was investigated by dual-culture assay. Strain CE 100 strongly inhibited mycelial growth of D. citri. The strain Bacillus subtilis KB-401 showed strong inhibitory effect on both mycelial growth and spore germination of D. citri (Nam et al., 2009). Similarly, in this study strain CE 100 was also found to inhibit the growth of D. citri.
Even though various kinds of biocontrol agents have been initiated as commercial products in the market, there are many limitations to their use such as the high cost of production (inoculum and low biomass productivity), development of stable formulation, variable biocontrol performance, and low efficacy in the field (Fravel, 2005; Pathak et al., 2017). The important factor for biomass production of these biocontrol agents is the cost effectiveness of the growth medium. According to Lewis (1991), a growth medium for liquid fermentation of a biocontrol strain should be developed based on inexpensive agricultural by-products. In the present study, a reliable method for large-scale cultivation of strain CE 100 has been introduced using the inexpensive growth medium. This medium has been optimized based on the fertilizers which are currently used by farmers in Korea. To attain better pathogen suppression, the population of the introduced biocontrol strain should be higher than those of native soil microorganisms (Ahmad et al., 2011). The population of inoculated biocontrol strain is often significantly lower than that of the indigenous microorganisms in the soil, resulting in low efficacy (Sylvia et al., 2005). Therefore, application of high density of biocontrol agent could dominate the native microbial community, thereby elevating their efficacy for successful pathogen inhibition. Therefore, our results in Fig. 2 and Fig. 3 indicate that adequate amounts of active metabolites and vegetative cells of B. velezensis CE 100 could be successfully produced in large-scale culture for onsite field application.
Several studies have shown that antagonistic bacteria are an important source of lytic enzymes that prevent the spread of fungal pathogens and reduce toxicity (Aktuganov et al., 2008; Kumar et al., 2011; Khabbaz et al., 2015). Fungal cell-wall degrading enzymes such as chitinase and β-1,3-glucanase of biocontrol bacteria, play a key role in the inhibition of fungal pathogens (Arora et al., 2007; Liu et al., 2011; Naing et al., 2014). Moon et al. (2021) demonstrated that B. velezensis CE 100 released lytic enzymes which were highly active in control of Phytophthora root rot of Chamaecyparis obtusa seedlings. Similarly, in the present study, we report production of different lytic enzymes, including chitinase and glucanase, which could be part of the inhibition mechanism employed by strain CE 100 based on the results of qualitative lytic enzyme assay. On the other hand, the BCFs of strain CE 100 from large-scale culture showed not only suppression of mycelial growth but also inhibition on spore germination of the target fungal pathogen. Treatment with these BCFs had a strong inhibitory effect on spore germination of D. citri (Table 1). This result seems to be associated with the action of lytic enzymes as well as of various antifungal metabolites in BCF. Choub et al. (2021) revealed the antifungal effect of the cyclic tetrapeptide, cyclo-(prolyl-valyl-alanyl-isoleucyl) which was isolated from the broth culture of B. velezensis CE 100, on mycelial growth of Colletotrichum gloeosporioides. In addition, as shown in Fig. 3, the degree of fungal inhibition on the plates augmented with BCF from large scale cultivation of strain CE 100 was similar when compared to BCF from small scale cultivation in TSB medium. These results indicate that there was no significant reduction in antifungal metabolite production of large-scale inoculation of strain CE 100 when compared to the small-scale (in lab) metabolite production in a laboratory grade medium. Microscopic observation also indicated twisted and vacuolated hyphal structures in the mycelium of D. citri treated with BCFs from large-scale culture (Fig. 4). Our results are in agreement with those of Maung et al. (2021), of which the mycelial growth of Botrytis cinerea was inhibited by different BCF concentrations of Bacillus amyloliquefaciens Y1 prepared from seven-day-old culture in fertilizer-based medium and hyphal structures with swelling and excessive branching of BCF treated mycelium were also examined under the light microscope.
Many studies have shown that microorganisms produce organic acids during fermentation to restrict other fast-growing bacterial and fungal species (Magnuson and Lasure, 2004). In our study, the pH of broth culture of strain CE 100 from large-scale cultivation was lower than those of small-scale cultures in the laboratory. The decrease in pH of broth culture of large-scale cultivation during early incubation period could be related to the secretion of organic acids that could be responsible for high inhibition of other mesophilic bacteria, thus preventing contamination.
In the report by Ko et al. (2014), inoculation with Pseudomonas putida THJ609-3 resulted in a remarkable reduction in melanose incidence on citrus leaves and inhibited spore germination of D. citri. The result of our pot experiment also demonstrated that melanose caused by D. citri on mandarin leaves was significantly reduced by strain CE 100 broth culture from large scale cultivation when compared to the control. Based on our results, the vegetative live cells and various antifungal metabolites secreted by strain CE 100 could be key players in controlling D. citri.
Conclusions
A cost-effective method for large-scale mass cultivation of strain CE 100 in an inexpensive PB medium was developed in our study and it can be easily applied in the field at lower cost, reducing that need for multiple dilutions. The results of our study also provide strong evidence that large scale inoculation of B. velezensis CE 100 was achieved with no growth of other undesirable microorganisms although no method was used to sterilize growth medium. In addition, the amount of enzymes and other active metabolites in the culture of strain CE 100 was sufficient to suppress D. citri under in vitro and in vivo conditions. We recommend further studies to isolate and identify active compounds from large-scale culture and its antimicrobial activity against different plant pathogens, thereafter, clarify the detailed mechanism behind fungal inhibition, and validate the consistent efficacy of these cultures in the field. Further experiment is also necessary to prove whether the organic acids produced by strain CE 100 play a major role or partial role in growth inhibition of other microorganisms.