Introduction
Materials and Methods
Prototypes containing illite, soil and fertilizer treatment
Growth conditions and sampling
Analysis of soil chemical properties and plant inorganic components
Extraction and measurement of chlorophyll and antioxidant capacity
Statistical analysis
Results and Discussion
Effect of illite-containing prototypes on soil chemical properties
Effect of illite-containing prototypes on lettuce growth
Effect of illite-containing prototypes on chlorophyll and antioxidant capacity of lettuce
Conclusions
Introduction
Crop plants obtain essential nutrients from soil, but soil nutrients are limited. Fertilizers are required to provide essential nutrients to crop plants and improve crop production and quality in modern agriculture (Isherwood, 2000). However, due to the limited nutrient use efficiency of plants, an excess supply of chemical fertilizers has resulted in environmental issues such as groundwater contamination by a leaching and/or runoff and air pollution by en emission. Therefore, the development and use of eco-friendly fertilizers are important to pursue both an improved fertilizer efficiency and reduced release of nutrients.
Clay minerals include inorganic ions such as K+, Ca2+ and Mg2+, which are a nutrient source for crop growth and development, in the interlayer space of particle (Kumari and Mohan, 2021). Despite of the relative lower cations-exchangeable capacity (CEC) compared to other clay minerals like perlite and vermiculite, illite possess higher K+ content to be available by plants (Weaver, 1965; Hashemi and Abbaslou, 2016; Paola et al., 2016). There are abundant illite to be used in industrial sectors including agriculture in Yeongdong-gun, Chungbuk province, South Korea (Cho et al., 2007). The main composition of illite is quartz (76%), illite (17%) and feldspar (7%) (Hwang et al., 2016). The effect of illite on soil amendement and crop growth promotion was reported (Barre et al., 2007; Lee et al., 2021), and thus it is required to find much evidence of the effect via extending into various crop plants.
Lettuce (Lactuca sativa L.) is one of leafy vegetables, which is being most extensively produced in the world. It is well known that lettuce shows an antioxidant capacity due to higher phenolic compounds, occupying more than 60% of total antioxidants (Nicolle et al., 2004). The potassium (K) fertilization in wheat production increased antioxidant capacity (Ahanger and Agarwal, 2017), and, additionally, it was reported that K fed by plants led to activate antioxidant metabolism (Ahanger et al., 2017). Illite-containing fertilizers showed a significant positive effect on improvement of soil quality including the effect of improving soil pH and plant growth promotion in tomato plants (Lee et al., 2021). The nutrient content and acidity of the soil can affect crop growth and nutrient content (Choi et al., 2021). Therefore, illite is considered as a valuable source to be used in agricultural area to pursue not only soil amelioration but healthy crop production. However, studies on the effect of illite have been conducted only on some crops, and thus it is required to prove an effect from various crop plants. Based on previous report (Lee et al., 2021), this study tried to develop a variety of illite-containing prototypes as alternative fertilizers and to examine their effects on the growth and antioxidative ability of lettuce plants.
Materials and Methods
Prototypes containing illite, soil and fertilizer treatment
In order to investigate the effects of illite-containing prototypes on pH amendement and plant growth promotion, two types of soil, acidic nutrient-poor soil (AC-NPS) and alkaline nutrient-rich soil (AK-NRS), were used in this work. The experimental soils, AC-NPS and AK-NRS, were obtained from the experiemtal fields of Chungbuk national university (Cheongju) and Galic research center (Danyang), respectively. The detailed information of soil including soil texture is described in Table 1. Illite, a clay mineral, was collected at Youngdong-gun, Chungbuk province (Table 2). Physicochemical properties of illite and three illite-containing prototypes were described in Table 3. The information on all types of illite-cotaining prototypes including the newly developed for the current work was shown in Table 3 and Fig. 1. Illite-containing prototypes are for soil amendment (prototype-A) and plant growth promotion (prototype-B and C). Both prototype-B and -C were manufactured with compound fertilizers, but the materials of the main inorganic fertilizers were different.
The treatments were composed as followed: no-fertilization, NPK, illite, prototype-A, prototype-B, and prototype-C. A dose of inorganic fertilizer (NPK) was determined from soil diagnosis (NIAS, 2017). Nitrogen (N, 188 kg ha-1), phosphorus (P, 215) and potassium (K, 149) were employed for the AC-NPS, and 74 (N) and 94 (P) were applied for the AK-NRS. The supply of K was ignored due to the surplus K content. Considering the relatively shorter cultivation period (30 days), urea (N), potassium phosphate (P) and potassium chloride (K) were applied as a basal dressing. Illite-containing prototypes were applied with 2,000 kg ha-1-equivalent as the recommended does of calcareous fertilizer which is a soil conditioner (NIAS, 2017). Inorganic fertilizer (NPK) was not entered into the illite-containing prototype treatments.
Table 1.
Physicochemical properties of experimental soils.
Properties | Unit | Appropriate† value | AC-NPS‡ | AK-NRS§ |
Soil texture | USDA | - | Sandy loam | Loam |
pH | 1:5, DW | 6.5 - 7.0 | 5.1 | 8.1 |
EC | 1:5, dS m-1 | ≤2 | 0.2 | 2.5 |
OM (TC × 1.724) | g kg-1 | 20 - 30 | 0.82 | 6.38 |
TN | wt% | - | 0.04 | 0.31 |
Avail. P2O5 | mg kg-1 | 250 - 400 | 4 | 304 |
Exch. K | cmolc kg-1 | 0.4 - 0.6 | 0.2 | 2.1 |
Exch. Ca | 6.0 - 7.0 | 0.6 | 21.3 | |
Exch. Mg | 2.0 - 2.5 | 0.9 | 4.6 | |
Exch. Na | - | 0.2 | 0.3 |
†Refer to the fertilizer use prescriptions standard (NIAS, 2017).
Table 2.
Physiocochemical composition of illite prototypes used in this study.
Physical properties | Mineral contents (%) | ||||||||
Thickness (Å) |
Diameter (nm) |
Base space (Å) |
Particle density (g cm-3) | SiO2 | Al2O3 | Fe2O3 | K2O | CaO | TiO2 |
30 | 0.1 - 0.3 | 10 | 2.6 | 57.5 | 23.3 | 6.76 | 6.43 | 0.1 | 0.38 |
Table 3.
Composition of various illite-containing prototypes.
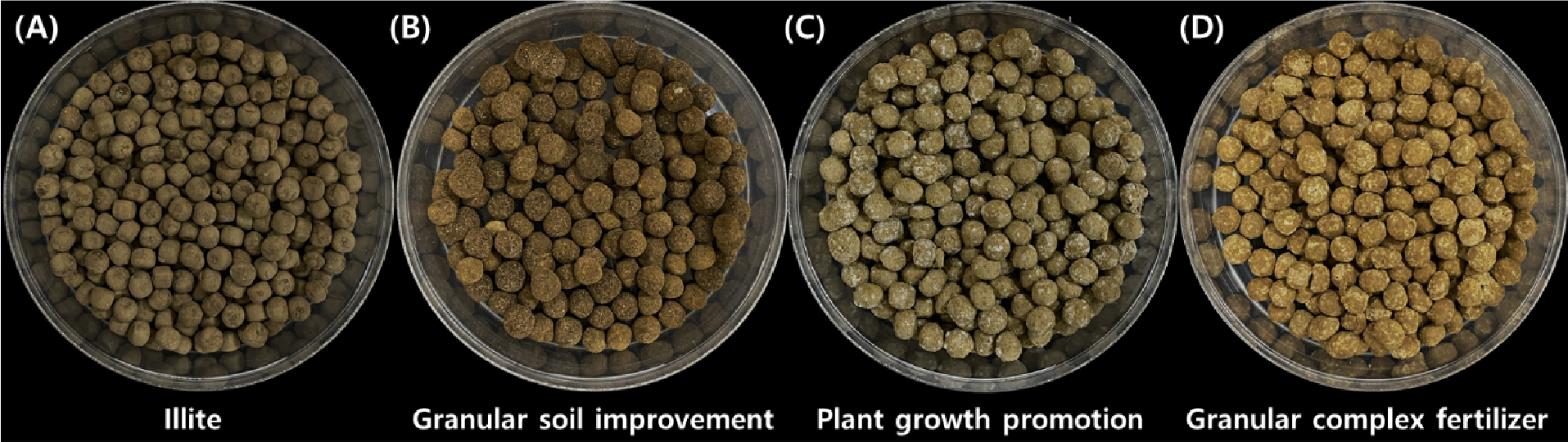
Fig. 1.
Different types of illite-containing prototypes for agricultural use. The detailed composition of each prototypes was described in Table 3.
Growth conditions and sampling
The seed of lettuce (Lactuca sativa L.) was germinated with a commercial nursery soil (128-hole plug tray), and uniformly grown lettuce seedlings (second leaf stage) were transplanted into plastic containers (20 cm width × 30 cm height, 10 kg of soil). Soil and plant samples for further analysis were carefully taken at 30 days after treatment. The treatment was arranged with the completely randomized plot design (n = 6). Leaf length and width were measured from the upper fully expanded leaves.
Analysis of soil chemical properties and plant inorganic components
Soil chemical properties were analyzed with an analytical manual (NIAS, 2000). The soil pH and electrical conductivity (EC) (soil: deionized-distilled (DD) H2O = 1:5) were measured with a pH and conductivity meter, respectively. Sample pH was measured using the FisherbrandTM accumetTM AB150 pH Benchtop Meters (Fisher Scientific, Loveland, CO, USA) which was calibrated with Thermo ScientificTM OrionTM buffer (Fisher Scientific, Loveland, CO, USA) with pH value of 4.01, 7.00 and 10.01. EC was measured using the Thermo ScientificTM Orion StarTM A212 Conductivity Benchtop Meter (Fisher Scientific, Loveland, CO, USA), precalibrated with 1,413 and 12,880 µS cm-1 obtained from Thermo Fisher Orion conductivity standard solution (Fisher Scientific, Loveland, CO, USA). Available phosphate was measured at 720 nm using a UV-visible spectrophotometer (UV 1900i, Shimadzu, Japan). Soil inorganic nitrogen (nitrate and ammonium) was measured with an automatic-nitrogen analyzer (440 nm, Auto analyzer 3, BRAN+LUEBBE, Germany) after extracting with 2 M KCl. Soil exchangeable cations including potassium (K), calcium (Ca) and magnesium (Mg) were measured using ICP (GBC, Integra XL, Australia) after the extraction with 2 M ammonium acetate buffer.
The concentrations of mineral nutrients were analyzed according to the standard method (Lee et al., 2017). The dried plant samples (0.5 g) were wet-digested with a solution (concentrated sulfuric acid including 5 mL of 368 mM salicylic acid), diluted with dd H2O and used as an analysis samples. Total N was measured with an automatic-nitrogen analyzer (440 nm, Auto analyzer 3, BRAN+LUEBBE, Germany), phosphorus was measured at measured at 470 nm (UV 1900i, Shimadzu, Japan) using a UV-visible spectrophotometer, and cations were measured using ICP (GBC, Integra XL, Australia).
Extraction and measurement of chlorophyll and antioxidant capacity
After the growth investigation, the above-ground lettuce parts were sampled and stored in a cryogenic freezer (-80°C) for analysis of chlorophyll and antioxidant activity. Chlorophyll analysis was followed: a ground fresh sample (0.5 g) was mixed with 50 mL 70% ethanol and stand at 4°C for 24 h. After extracting 2 mL from the mixture, 8 mL acetone was added and measured absorbance at 645 and 663 nm. These values were then used in the calculation of the following (Porra, 2002) :
Total polyphenols and flavonoids content were analyzed for antioxidant capacities. The 2,2′-azino-bis-3-ethylbenzthiazoline-6-sulphonic acid (ABTS) and 1,1-diphenyl-2-picrylhydrazyl (DPPH) radical were measured to evaluate radical scavenging. To measure the antioxidant capacities, the fresh sample was extracted: the ground sample powder (0.5 g) was mixed with 70% ethanol (15 mL) and stirred at 200 rpm for 30 min. After centrifugation and filtration (0.45 µm), the extraction was used analysis.
Total polyphenol was determined using Folin-Ciocalteu’s phenol reagent (Folin and Denis, 1912). The reaction mixture including 250 µL of extraction, 5 mL of 2% Na2CO3 and 250 µL of Foline-Ciocalteu reagent reacted in the dark for 30 minutes, and was measured at 750 nm. Gallic acid was used as a standard. Total polyphenol was quantified according to the calibration curve (y = 0.001x + 0.0021, R2 = 0.999) in the range of gallic acid 0 - 100 µg mL-1.
Total flavonoid was measured with Moreno method (Moreno et al., 2000). Extraction solution (500 µL) was mixed with 150 µL of NaNO2 and 2 mL of deionized-distilled water and stand for 5 min at RT. The 300 µL of 10% AlCl3 ‧ 6H2O was added into the reaction mixture and reacted for 6 min. After an addition of 1 mL of 1 M NaOH, the reaction mixsture reacted for 11 min at RT and was measured at 510 nm. Catechin was used as a standard. Total flavonoid was quantified according to the calibration curve (y = 0.0055x + 0.0001, R2 = 0.999) in the range of catechin 0 - 100 µg mL-1.
For the ABTS assay, 7.4 mM ABTS was dissolved in 2.6 mM K2SO4. The reaction buffer was incubated for one day at RT in the dark and diluted until the value of absorbance measured 1.0 at 735 nm. According to Re method (Re et al., 1999), the extract (200 µL) was mixed with 4 mL of the reaction solution and reacted for 30 min in the dark. The mixture was measure at 735 nm. The percentage of ABTS scavenging capacity was calculated using the formula:
For the DPPH assay, 0.2 mM DPPH was dissolved in ethanol. The measurement was followed by the Blois method (Blois, 1958). The extract (750 µL) and 0.2 mM DPPH solution were mixed and reacted for 30 minutes at RT in the dark, and was measured at 525 nm. The percentage of DPPH scavenging capacity was calculated as:
Statistical analysis
Statistical analysis was conducted using SAS software version 9.2 (SAS Institute, Cary, NC, USA). Data were compared using one-way ANOVA and LSD test. And graphs were made using Sigma Plot (v. 14.5, SYSTAT, Palo Alto, CA, USA).
Results and Discussion
Effect of illite-containing prototypes on soil chemical properties
All prototype of illite-containing fertilizers in AC-NPS significantly increased the pH of soil by 0.4 to 0.9 compared to before the test and without fertilizer (Table 4). In contrast, soil pH in AK-NRS did not show the similar patterns between illite-only and illite-containing prototypes. The electrical conductivity (EC), a soil nutrient index (Mazur et al., 2022), was significantly elevated by an addition of illite-containing prototypes in AC-NPS, whereas the higher EC in AK-NRS was only observed in the prototype-A. Total nitrogen (N) in soil showed a significantly increasing tendency, and this was most remarkable in the prototype-A in both AC-NPS and AK-NRS. On the other hand, soil K was the most abundant in the prototype-B and -C in both soil types, and soil calcium (Ca) was greatly enhanced in AC-NPS. Our result was in line with previous observations (Lee et al., 2012, 2021), which the soil potassium (K) and calcium (Ca) were markedly increased by the application illite-containing materials. Taken together, the relative abundant cations of illite contributed to an enhancement of soil potassium (K) and calcium (Ca), and this suggests that illite-containing prototypes could be used as a nutrient provider for crop growth and development.
Table 4.
Soil chemical properties by application of different illite-containing prototypes. Data indicate mean ± S.D. (n = 3).
Soil† | Time‡ | Treatment |
pH (1:5) |
EC (dS m-1) |
T-N (%) |
Avail. P2O5 (mg kg-1) | Exch. cations (cmolc kg-1) | ||
K | Ca | Mg | |||||||
AC- NPS | Before | - | 5.1 ± 0.0 c§ | 0.2 ± 0.0 f | 0.04 ± 0.00 f | 4 ± 0 e | 0.2 ± 0.0 bc | 0.6 ± 0.0 e | 0.9 ± 0.0 a |
After | No fert. | 5.1 ± 0.1 c | 0.2 ± 0.0 e | 0.47 ± 0.02 c | 4 ± 1 e | 0.2 ± 0.0 d | 0.8 ± 0.0 d | 0.6 ± 0.0 d | |
NPK | 5.9 ± 0.0 a | 0.3 ± 0.0 d | 0.42 ± 0.03 de | 17 ± 1 c | 0.2 ± 0.0 d | 1.1 ± 0.0 b | 0.7 ± 0.0 c | ||
Illite | 5.5 ± 0.3 b | 0.3 ± 0.0 d | 0.45 ± 0.02 cd | 3 ± 0 e | 0.2 ± 0.0 cd | 1.1 ± 0.0 b | 0.8 ± 0.0 b | ||
Prototype-A | 5.8 ± 0.0 a | 0.3 ± 0.0 c | 0.54 ± 0.01 a | 8 ± 1 d | 0.2 ± 0.0 cd | 1.0 ± 0.0 c | 0.6 ± 0.0 d | ||
Prototype-B | 5.8 ± 0.1 a | 0.5 ± 0.0 b | 0.50 ± 0.02 b | 26 ± 1 a | 0.3 ± 0.0 a | 1.3 ± 0.0 a | 0.8 ± 0.0 b | ||
Prototype-C | 6.0 ± 0.0 a | 0.7 ± 0.0 a | 0.42 ± 0.03 e | 23 ± 0 b | 0.3 ± 0.0 ab | 1.0 ± 0.0 c | 0.8 ± 0.0 d | ||
AK- NRS | Before | - | 8.1 ± 0.0 d | 2.5 ± 0.1 b | 0.31 ± 0.04 f | 304 ± 53 c | 2.1 ± 0.0 a | 21.3 ± 0.2 a | 4.6 ± 0.1 a |
After | No fert. | 8.3 ± 0.0 b | 2.5 ± 0.0 b | 3.78 ± 0.08 b | 432 ± 9 b | 1.4 ± 0.0 c | 13.8 ± 0.1 c | 3.8 ± 0.0 c | |
NPK | 8.3 ± 0.0 b | 2.1 ± 0.0 c | 3.69 ± 0.11 bc | 455 ± 17 ab | 1.5 ± 0.1 bc | 13.3 ± 0.2 d | 3.7 ± 0.1 c | ||
Illite | 8.4 ± 0.1 a | 1.5 ± 0.0 d | 3.44 ± 0.04 e | 419 ± 11 b | 1.4 ± 0.0 c | 12.1 ± 0.1 f | 3.3 ± 0.1 e | ||
Prototype-A | 8.1 ± 0.1 cd | 2.9 ± 0.1 a | 3.91 ± 0.11 a | 473 ± 6 a | 1.4 ± 0.0 c | 14.5 ± 0.1 b | 3.9 ± 0.0 b | ||
Prototype-B | 8.2 ± 0.0 b | 2.5 ± 0.0 b | 3.52 ± 0.06 de | 474 ± 4 a | 1.4 ± 0.0 bc | 12.6 ± 0.2 e | 3.5 ± 0.1 d | ||
Prototype-C | 8.2 ± 0.0 bc | 2.5 ± 0.0 b | 3.59 ± 0.03 cd | 487 ± 8 a | 1.5 ± 0.1 b | 12.6 ± 0.1 e | 3.5 ± 0.1 d |
Effect of illite-containing prototypes on lettuce growth
Indeed, the level of mineral nutrients affects plant growth and development. Number of lettuce leaf was significantly much more in AK-NRS compared to AC-NPS, which indicated approximately 2 to 3-fold higher (Table 5). Chemical fertilizer in AC-NPS resulted in distinct greater leaf development, and this contributed to significant increase in leaf fresh weight. In contrast, eventhough much more leaf development compared to AC-NPS, the AK-NRS-grown lettuce did not show significant differences in leaf development and fresh weight among treatments. However, illite-containing prototypes in AC-NPS extremely promoted an increase in leaf length, and it was obvious by prototype-B and -C. Leaf width also showed an increasing tendency by an application of illite-containing prototypes, although there was not significant difference among treatment groups. This result was in line with our previous study with tomato, which indicated that illite-containing fertilizer showed relatively lower growth rate compared to inorganic fertilizer (Lee et al., 2021). From the result, at least, illite-containing prototypes have an effect on the size of an individual leaf rather than number of leaf.
Table 5.
Leaf parameter and growth of lettuce plants fed with different illite-containing prototypes for 30 days. Data indicate mean ± S.D. (n = 3).
Soil† | Treatment |
No. of leaves (per plant) |
Leaf length (cm) |
Leaf width (cm) |
Fresh weight (g plant-1) |
AC-NPS | Control | 7.3 ± 1.5 b‡ | 10.1 ± 0.5 b | 4.6 ± 0.2 b | 3.2 ± 1.5 b |
NPK | 12.0 ± 0.0 a | 15.3 ± 0.5 a | 6.3 ± 1.1 a | 6.5 ± 0.3 a | |
Illite | 7.3 ± 1.5 b | 7.3 ± 0.8 c | 4.5 ± 0.3 b | 3.4 ± 0.7 b | |
Prototype-A | 9.3 ± 0.6 b | 11.1 ± 1.2 b | 5.0 ± 0.1 b | 4.7 ± 0.5 ab | |
Prototype-B | 7.0 ± 1.0 b | 10.8 ± 1.3 b | 5.1 ± 0.6 b | 4.0 ± 1.3 b | |
Prototype-C | 8.7 ± 2.5 b | 7.1 ± 0.8 c | 4.6 ± 0.5 b | 4.3 ± 1.5 b | |
AK-NRS | Control | 23.0 ± 1.7 ab | 17.6 ± 1.7 bc | 8.7 ± 1.1 ns | 21.5 ± 3.7 ns |
NPK | 23.7 ± 2.9 a | 16.1 ± 2.2 c | 9.2 ± 1.4 | 19.3 ± 4.5 | |
Illite | 23.0 ± 2.6 ab | 15.5 ± 2.8 c | 9.0 ± 1.5 | 21.5 ± 1.4 | |
Prototype-A | 21.0 ± 2.0 ab | 17.1 ± 1.1 bc | 10.5 ± 0.5 | 17.8 ± 4.4 | |
Prototype-B | 19.3 ± 2.1 b | 22.0 ± 2.4 a | 10.2 ± 2.1 | 16.1 ± 3.9 | |
Prototype-C | 20.7 ± 1.5 ab | 20.5 ± 3.4 ab | 11.1 ± 1.7 | 18.2 ± 2.3 |
Macro nutrients including N was also measured at 30 DAT from the shoot (leaves) of lettuce grown under different soil and fertilization types (Table 6). Except for total carbon (T-C), all measured nutrients were significantly higher in AC-NPS-grown lettuce. In both soil types, the prototype-B application resulted in the highest N concentration, 4.76 (AC-NPS) and 6.10 (AK-NRS), respectively. In particular, illite-containing prototypes greatly contributed to the accumulation of leaf N in AC-NPS, and, promoted the significant increase in K, Ca and Mg. The prototype-B also showed the similar effect in AK-NRS, which enhanced the level of T-N, Ca and Mg. This is the similar to the result that illite treatment increased mineral content of bean sprouts (Ha et al., 2022). The T-C was significantly higher in the AC-NPS-grown lettuce compared to those in AK-NRS, and it is closely related to N content. The absorbed N is preferentially used for the production of nitrogen-containing organic compounds such as amino acids and chlorophyll (Ohyama, 2010). The previous studies well documented that the N supply led to an abundant N content in leaves, which is mainly located in the photosynthetic protein complex, thereby enhancing photosynthesis and plant growth (Vos et al., 2005; Liu et al., 2019; Yue et al., 2021). Therefore, our observation suggests that the prototype-B could be an useful provider to promote nutrient uptake by lettuce plant, and an availability of nutrients is not affected by soil chemical properties.
Table 6.
Macro nutrients of lettuce (Lactuca sativa L.) plants applied with different types of illite-containing agricultural prototypes (n = 3).
Soil† | Treatment |
T-C (%) |
T-N (%) |
P2O5 (%) |
K2O (%) |
CaO (%) |
MgO (%) |
AC- NPS | Control | 40.5 ± 0.1 a‡ | 1.52 ± 0.03 d | 0.16 ± 0.02 cd | 4.12 ± 0.15 c | 0.34 ± 0.01 c | 0.24 ± 0.01 d |
NPK | 40.1 ± 0.1 b | 2.31 ± 0.03 c | 0.17 ± 0.01 c | 4.42 ± 0.35 c | 0.49 ± 0.04 ab | 0.28 ± 0.02 b | |
Illite | 39.7 ± 0.2 c | 2.16 ± 0.07 c | 0.17 ± 0.01 c | 4.54 ± 0.18 c | 0.51 ± 0.02 a | 0.29 ± 0.01 ab | |
Prototype-A | 40.6 ± 0.2 a | 2.08 ± 0.07 c | 0.15 ± 0.01 d | 4.23 ± 0.33 c | 0.46 ± 0.01 b | 0.27 ± 0.02 bc | |
Prototype-B | 39.9 ± 0.2 bc | 4.76 ± 0.43 a | 0.27 ± 0.01 a | 5.75 ± 0.22 a | 0.51 ± 0.02 a | 0.32 ± 0.02 a | |
Prototype-C | 40.4 ± 0.2 a | 3.37 ± 0.14 b | 0.19 ± 0.01 b | 5.11 ± 0.28 b | 0.36 ± 0.02 c | 0.25 ± 0.01 cd | |
AK- NRS | Control | 36.0 ± 0.1 b | 5.84 ± 0.01 bc | 0.65 ± 0.01 ns | 10.06 ± 0.48 a | 1.83 ± 0.07 b | 0.75 ± 0.04 ab |
NPK | 36.0 ± 0.2 b | 5.77 ± 0.05 c | 0.64 ± 0.02 | 9.35 ± 0.23 abc | 1.87 ± 0.08 b | 0.68 ± 0.02 ab | |
Illite | 37.0 ± 0.1 a | 5.35 ± 0.08 d | 0.65 ± 0.02 | 9.21 ± 0.59 bc | 1.55 ± 0.09 c | 0.56 ± 0.02 c | |
Prototype-A | 35.9 ± 0.2 b | 6.06 ± 0.14 a | 0.64 ± 0.01 | 8.68 ± 0.16 c | 1.92 ± 0.08 ab | 0.64 ± 0.03 bc | |
Prototype-B | 34.8 ± 0.1 c | 6.10 ± 0.04 a | 0.65 ± 0.01 | 9.71 ± 0.20 ab | 2.13 ± 0.16 a | 0.81 ± 0.06 a | |
Prototype-C | 35.0 ± 0.2 c | 5.92 ± 0.08 b | 0.68 ± 0.01 | 9.29 ± 0.56 abc | 2.09 ± 0.18 a | 0.74 ± 0.04 a |
Effect of illite-containing prototypes on chlorophyll and antioxidant capacity of lettuce
The content of chlorophyll a and b was obviously higher in lettuce treated with the prototype-B and -C in AK-NRS, even although, in AC-NPS, chemical fertilization (NPK) showed the greatest level (Fig. 2). By contrast, chlorophyll a/b ratio did not differ from the treatments, both soil and fertilization. Compared to T-N content of lettuce leaves, there was no or little relationship between N and chlorophyll contents. It seems that nitrogen is preferentially metabolized into chlorophyll biosynthesis pathway to promote photosynthesis, as observed in previous study which indicated three times greater in tomato plant by an application illite-containing fertilizer (Lee et al., 2021). Based on our result, the prototype-B and -C clearly represent an enhancing effect of chlorophyll production in the AC-NPS-grown lettuce plant.
The antioxidant activity such as total polyphenols, total flavonoids, ABTS+ and DPPH were observed from the lettuce leaves treated with different soil and fertilization types (Fig. 3). All types of antioxidative indicators significantly greater in the AC-NPS-grown lettuce leaves irregardless of the types of fertilization including illite-containing prototypes. Additionally, the antioxidant activity resulted in an inverse proportion with fertilization levels. In particular, in AC-NPS, illite was significantly increased content of total polyphenols (19.5 mg g-1), flavonoids (3.8 mg g-1), and ABTS scavenging capacity (37.2%). This is the similar pattern to previous observations with tomato and soybean (Lee et al., 2021; Ha et al., 2022). Therefore, antioxidant activity is greatly depended on the level of available nutrients in soil due to the relatively higher nutrient of illite-containing prototypes.
Conclusions
In conclusion, this work tried to examine whether various illite-containing prototypes as an alternative chemical fertilizers are the effects on lettuce growth, nutrient uptake and antioxidant activity. Of those, prototype-B and -C elevated EC value in the AC-NPS. Illite-containing prototypes showed an enlargement of individual leaf of AK-NRS-grown lettuce plant. Additionally, prototype-B and -C resulted in an enrichment of macro nutrients (N, K and Ca) and antioxidant activity in the AC-NPS. Taken together, illite-containing prototypes (B and C) could be used as an alternative or supplement of chemical fertilizer for suitable lettuce grown in the AC-NPS. On the basis of positive effects of illite-containing prototype fertilizer, further study is suggested to be extend to various vegetable crops.