Methane emission from soil surface during rice growth season under different temperature, fertilization, and rice straw input conditions
ABSTRACT
Introduction
Materials and Methods
벼 재배 실험
메탄 포집 및 분석
통계 분석
Results and Discussion
Conclusions
Introduction
농업 분야의 2020년 온실가스 배출량은 국가 총 배출량의 3.2%에 해당하는 2,105만 톤으로, 전년보다 9만 톤 (0.4%)이 증가하였다. 농업 분야 온실가스 배출 경로는 장내 발효, 가축분뇨, 농경지 토양, 벼 (Oryza sativa L.) 재배, 작물잔사 소각으로 구분된다 (GIR, 2022). GIR (2022)에 따르면, 벼 재배 부문이 농업 분야 온실가스 배출 중 27.1%로 가장 높은 비중을 차지하며, 벼 재배 면적 감소에 따라 전년 대비 22만 톤 (3.6%) 감소하였다. 하지만, 논은 여전히 온실가스인 메탄의 주요 발생원으로 작용하고 있다 (Baek et al., 2023; Lee et al., 2023). 논 토양에서 생성된 메탄은 벼 식물체의 통기 조직을 통한 운송, 토양 표층을 통한 불규칙적 분출, 그리고 대기와의 농도 차이에 의한 확산을 주요 경로로 대기로 방출된다 (Gwon et al., 2022). 주요 세 가지 경로 중 논에서 방출되는 메탄의 90% 이상이 벼의 통기 조직을 통해 대기로 방출되는 것으로 알려져 있어 (NAAS, 2013), 대부분의 연구에서는 이를 주요하게 고려한다 (Seo et al., 2011; Ju et al., 2013; Kim et al., 2016; Jeong et al., 2018). 비록, 논에서 메탄 플럭스를 측정할 때 벼와 함께 토양 표면도 일정 면적 포함되지만, 토양에서 분출과 확산 등을 통해 직접 방출되는 메탄에 대한 연구는 부족하다 (Tokida et al., 2013).
일부 선행 연구에서 분출에 의한 메탄 방출을 조사하였는데 (Green, 2013; Tokida et al., 2013; Kajiura and Tokida, 2021), 벼를 포함한 전체 메탄 방출에 대한 분출을 통한 메탄 방출 비율은 유수형성기에는 26 - 45%, 출수기에는 60 - 68%로 매우 높은 수준으로 보고된 바 있다 (Tokida et al., 2013). 하지만, Tokida et al. (2013)의 연구에서는 벼 뿌리를 포함한 벼 식물체는 그대로 두고 벼 식물체 지상부만 제거한 후 토양직접방출 메탄을 측정하여 잔존 벼 분얼의 통기 조직을 통한 메탄 방출 가능성을 배제하지 못하는 한계가 있다고 보고하였다. 따라서, 토양직접방출 메탄을 평가하기 위해서는 벼 식물체가 없는 토양 표면에서의 메탄 측정 연구가 필요하다.
논에서 메탄은 메탄생성균의 유기물의 혐기적 분해에 의해 발생되기 때문에 (Malyan et al., 2016) 기상과 시비 등의 요인에 의해 영향을 받을 수 있는데, 벼 생장 여건이 양호하여 벼 생장이 증가할 경우 뿌리를 통한 유기물 분비에 의해 메탄 생성량이 증가할 수 있다 (Gwon et al., 2019; Lee et al., 2020b). 특히, 기온 상승 조건에서 벼의 분얼수가 증가하고 생체량이 증가하여 뿌리 삼출물이 많아지면 메탄 생성량이 증가하는 것으로 보고된 바 있다 (Sass et al., 1990). 하지만, 토양에서 생성된 메탄이 대기로 이동할 수 있는 통기조직 (즉, 분얼수)도 같이 증가하여 토양직접방출 메탄에는 변화가 없을 수도 있다 (가설 1). 한편, 화학비료를 사용하는 관행논에 비해 볏짚과 유기질 비료, 퇴비 등 유기물을 투입하는 유기농에서 메탄 생성량이 1.4 - 1.9배 증가한다고 보고된 바 있다 (Kim et al., 2002). 이와 같은 조건에서는 벼 통기 조직 바이오매스에는 변화가 없는 조건에서 토양에서 생성되는 메탄이 많아질 경우 벼의 통기 조직이 증가된 메탄을 모두 수용할 수 없어 토양직접방출 메탄이 증가할 가능성이 있다 (가설 2). 하지만, 기온 상승과 유기물 투입 여부에 따른 토양직접방출 메탄에 대한 연구는 매우 제한적이다. 따라서, 본 연구에서는 논에서의 메탄 방출 경로에 대한 이해를 확대하기 위해 위의 두 가지 가설을 설정하여 벼 생육기간 중 온도가 상이한 조건에서 화학비료와 볏짚 또는 유기질 비료 + 퇴비 투입조건에서 토양직접방출 메탄을 조사하였다.
Materials and Methods
벼 재배 실험
본 연구는 광주광역시 용봉동에 위치한 전남대학교 실험 포장 (35°10'26.6"N, 126°53'53.9"E)에 설치된 Temperature Gradient Chambers (TGCs)를 이용하여 수행하였다. TGCs의 온도 제어 방식은 선행 연구에 충분히 설명되어 있다 (Kim et al., 2011; Nam et al., 2013; Park et al., 2020). 벼는 선행 연구 (Kim et al., 2011; Nam et al., 2013)와 동일한 방식으로 포트 (지름 30 cm, 깊이 38 cm, 토양 20 kg) 재배하였다. 온도, 시비 처리, 볏짚 투입 여부를 달리한 12개의 처리구를 3반복하여 36개의 포트로 벼 재배 실험을 수행하였다.
온도는 대기 온도와 상승 온도 (+3°C) 조건으로 설정하였으며, 시비 처리는 무처리, 화학비료, 유기질 비료 + 퇴비 처리구를 두었다. 화학비료, 유기질 비료와 퇴비는 모내기 1주일 전에 표층 5 cm와 혼합하였다. 화학비료 시비구에는 CO(NH2)2, KH2PO4, KCl로 N-P2O5-K2O를 9.0-4.5-5.7 g m-2 투입하였으며, 유기질 비료 + 퇴비 시용구에는 유박 (332.5 g C kg-1, 60.3 g N kg-1, 5.4 g P kg-1, 13.1 g K kg-1)을 화학비료 시비구의 질소 시비량과 동일하게 포트 당 10.6 g (건조 기준) 투입하였고, 퇴비 (224.4 g C kg-1, 22.5 g N kg-1, 10.8 g P kg-1, 13.2 g K kg-1)는 우리나라에서 일반적으로 권장되는 1.5 kg m-2 수준으로 포트 당 106 g (건조 기준) 투입하였다. 유기질 비료와 퇴비 투입에 의해 인산과 가리는 각각 39.0 g m-2과 26.2 g m-2이 추가 공급되었다. 볏짚 처리구에는 전년도에 발생한 볏짚의 90% (50 - 141 g pot-1)를 당해 년도 모내기 60일 전에 토양 10 cm와 혼합하여 투입하였다. 온도와 시비 조건에 따라 볏짚 생산량이 처리별로 달라서 투입된 볏짚의 양도 달랐다. 볏짚 (탄소 함량 약 400 g C kg-1) 처리에 의해 약 20 - 56 g의 탄소가 투입된 반면, 유박과 퇴비 투입에 의해서는 각각 3.5 g과 24 g의 탄소가 투입되었다. 따라서, 일부 볏짚 처리구에서는 볏짚 투입과 상응하는 양의 탄소가 퇴비로 투입되었다.
2022년 5월 30일에 30일생 벼 유묘 (품종 새청무) 3포기를 포트 토양에 심고, 모내기 후 41 - 48일 간의 중간낙수기와 벼 수확 1주일 전을 제외하고 매일 관개수를 공급하여 담수위를 3 - 5 cm로 유지하여 벼를 재배하였으며, 9월 28일에 수확하였다.
메탄 포집 및 분석
벼 재배 과정 중 중간 낙수를 7월 12 - 20일에 실시하였고, 이를 기준으로 중간낙수 전 (7월 6일), 중간낙수 기간 (7월 20일), 중간낙수 후 (8월 11일)에 벼를 포함한 메탄 플럭스와 토양직접방출 메탄 플럭스를 3회 측정하였다.
벼를 포함한 메탄 포집용 챔버 (지름 40 cm, 높이 110 cm)와 토양직접방출 메탄 포집용 챔버 (지름 5 cm, 높이 20 cm)는 아크릴로 제작하였다. 벼 포함 메탄 포집은 관행적인 방법으로 수행하였으며 (Minamikawa et al., 2015), 토양직접방출 메탄 플럭스는 각 처리구 포트 내부에서 벼를 포함하지 않는 토양 표면에서 5 cm 깊이로 챔버를 설치하고 환기를 위해 Septa를 열어놓고 4시간 이상 토양 안정화를 시켜주었다 (Fig. 1). 가스는 Septa를 닫고 10분 경과 후 주사기 (5 mL)로 1차 채취하였으며, 60분경과 후 2차로 채취하여 진공바이얼 (4 mL)에 옮겼다.
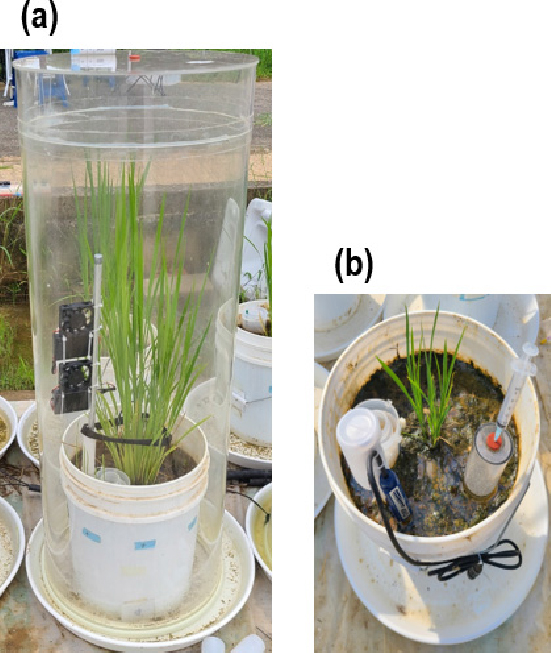
Fig. 1.
Methods for gas samplings: (a) for whole rice plants and (b) for soil surface only.
진공바이얼에 포집된 가스 시료 1 mL 를 가스크로마토그래피 기기 (Agilent 7890A GC, Agilent, CA, USA)를 이용해 분석하였으며, 메탄 배출량은 다음의 Eq. 1로 계산하였다 (Jeong et al., 2018).
: CH4 flux (mg m-2 hr-1)
: gas density (mg m-3)
: volume of chamber (m3)
: surface area of chamber (m2)
: rate of increase of gas concentration (mg m-3 hr-1)
: absolute temperature (273 + mean temperature in chamber) (°C)
통계 분석
생육 시기별 온도, 시비, 볏짚 투입에 따른 토양직접방출 메탄 플럭스 변화의 통계적 유의성은 IBM SPSS Statistics 27 (IBM Corp., Amonk, New York, USA) 프로그램을 이용하여 유의성 95% 수준으로 분산분석하여 검토하였고, 분산분석이 유의할 경우 Duncan’s multiple range test로 사후 분석하였다.
Results and Discussion
벼 생육시기별로 측정한 메탄 플럭스 전체에 대해 온도, 시비 처리, 볏짚 투입 여부에 따른 벼를 포함한 단위면적당 메탄 플럭스와 토양직접방출 메탄 플럭스 모두 차이가 없었다 (Table 1). 일반적으로 메탄 생성량은 벼의 생체량이 많아지면 증가하는 경향이기 때문에 토양직접방출 메탄 플럭스도 증가할 수 있다 (Sass et al., 1990). 따라서, 상승 온도 조건에서 화학비료 또는 유기질 비료를 시용하고 볏짚을 혼합한 처리구에서 벼를 포함한 단위면적당 메탄 플럭스와 토양직접방출 메탄 플럭스도 증가할 수 있지만, 본 연구에서는 이와 같은 결과를 확인하지 못했다. 이는 벼 생체량이 많아지면 분얼수도 같이 증가하게 되는데, 벼의 통기 조직이 메탄이 방출 통로로는 기능하지 않고 외기 산소 (O2)의 확산 경로로도 활용되기 때문에 혐기적 조건에서 생성된 메탄이 근권 주변의 호기적 조건에서 산화되어 메탄 플럭스에는 변화가 없을 수도 있음을 의미한다 (Denier van der Gon et al., 1996; Kim et al., 2017; Bhattacharyya et al., 2019; Wang et al., 2019). 한편, 토양직접방출 메탄 플럭스의 경우, Tokida et al. (2007)이 언급한 바와 같이 토양에서 분출 등을 통한 메탄 방출은 토양 표면에서 균일하고 지속적으로 일어나지 않고 간헐적이면서 비균질적으로 발생하기 때문에 본 연구에서 토양 표면 일부에서 측정한 토양직접메탄 방출의 대표성에 문제가 있을 수도 있다.
Table 1.
Probability (P > F) values for the analysis of variance on methane flux per unit area including rice (total flux) and direct methane flux from soil (soil flux).
Treatments
|
Probability > F |
Total (rice + soil) flux
|
Soil flux
|
Temperate
|
0.860
|
0.363
|
Fertilizer
|
0.278
|
0.486
|
Straw
|
0.018
|
0.514
|
Time
|
0.150
|
0.521
|
Temperate × Fertilizer
|
0.425
|
0.102
|
Temperate × Straw
|
0.181
|
0.330
|
Temperate × Time
|
0.385
|
0.143
|
Fertilizer × Straw
|
0.228
|
0.283
|
Fertilizer × Time
|
0.132
|
0.793
|
Straw × Time
|
0.000
|
0.446
|
Temperate × Fertilizer × Straw
|
0.796
|
0.124
|
Temperate × Fertilizer × Time
|
0.313
|
0.306
|
Temperate × Straw × Time
|
0.359
|
0.216
|
Fertilizer × Straw × Time
|
0.804
|
0.584
|
Temperate × Fertilizer × Straw × Time
|
0.342
|
0.368
|
한편, 벼 생육 시기 별 메탄 플럭스를 비교하면, 벼 생장 초기에 해당하는 중간낙수 전의 토양직접방출 메탄 플럭스는 볏짚 투입에 의해 증가하였다 (Table 2). 벼를 포함한 단위면적당 메탄 플럭스와 토양직접방출 메탄 플럭스 전체 평균은 각각 20.7 mg m-2 hr-1와 0.22 mg m-2 hr-1로 토양직접방출 메탄 플럭스는 벼를 포함한 단위면적당 메탄 플럭스의 약 1% 수준이었다 (Figs. 2 and 3). 본 연구에서 토양직접방출 메탄의 비율이 Tokida et al. (2013)의 연구결과에 비해 매우 낮은데, 이는 Tokida et al. (2013)의 연구에서는 벼 식물체 지상부를 제거한 후 토양직접방출 메탄을 측정하여 남아있는 벼 분얼 조직을 통한 메탄 방출도 포함되었기 때문으로 판단된다.
비록 통계적 유의성은 확인되지 않았지만 (Tables 1 and 2), 벼를 포함한 단위면적당 메탄 플럭스 평균은 볏짚 투입구 (25.13 mg m-2 hr-1)에서 무투입구 (16.49 mg m-2 hr-1)보다 높은 경향이었으며 (Fig. 2), 토양직접방출 메탄 플럭스 평균 또한 볏짚 투입구 (0.35 mg m-2 hr-1)에서 무투입구 (0.1 mg m-2 hr-1)보다 높은 경향이었다 (Fig. 3). 특히, 앞에서 언급한 바와 같이 벼 생육 초기인 중간낙수 전에 볏짚 투입에 의해 메탄 발생량이 크게 증가하였는데 이는 볏짚이 메탄생성균의 기질로 활용되었기 때문이다 (Gwon et al., 2022). 특히, 전체 메탄 방출량에 대한 토양직접방출 메탄의 비율이 벼 생육 초기인 중간낙수 전 볏짚투입구에서 약 5%이었고, 볏짚 무투입구에서 약 1.5%로 벼 생육 초기에 볏짚 투입에 의해 토양직접방출 메탄도 증가하였다 (P = 0.001) (Fig. 4, Table 3). 하지만, 중간낙수기간에는 볏짚 투입 여부에 따른 영향이 나타나지 않았고 중간낙수기 이후에 다시 볏짚 투입구에서 토양직접방출 메탄이 증가하는 경향이었다 (Fig. 4). 이는 담수 초기에는 볏짚이 빠르게 분해되고, 벼 통기조직은 미발달되어 통기조직의 메탄 수용력이 낮은 조건에서 과잉의 메탄이 분출과 확산을 통해 토양 표면을 통해 직접 배출되기 때문으로 판단된다. 논에 투입되는 대표적인 유기물인 볏짚에 의해 논의 메탄 배출량은 볏짚 무투입에 비해 최대 200% 이상 증가하며, 특히 볏짚 투입 초기 담수 조건에서 메탄 발생량이 크게 높아지는 것으로 보고되고 있다 (Wang et al., 2012; Zhao et al., 2015; Lee et al., 2020a). 또한, 중간낙수기 이후 볏짚 투입에 의해 토양직접방출 메탄이 다시 증가한 것은 중간낙수기 동안 볏짚의 난분해성 성분의 추가적인 분해에 의한 메탄 기질 공급에 의한 것으로 판단된다 (Park et al., 2020). 따라서, 본 연구 결과에 의하면 토양직접방출 메탄은 유기물에 따른 메탄 발생 시기와 벼 생육단계에 따른 통기조직을 통한 메탄 이동에 의해 영향을 받는 것으로 나타났다.
Table 2.
Probability values for the analysis of variance on direct methane flux from soil in different season.
Time1 |
Treatments
|
Probability > F |
T1
|
Temperate
|
0.247
|
Fertilizer
|
0.142
|
Straw
|
0.001
|
Temperate × Fertilizer
|
0.119
|
Temperate × Straw
|
0.587
|
Fertilizer × Straw
|
0.071
|
Temperate × Fertilizer × Straw
|
0.088
|
T2
|
Temperate
|
0.421
|
Fertilizer
|
0.418
|
Straw
|
0.744
|
Temperate × Fertilizer
|
0.178
|
Temperate × Straw
|
0.518
|
Fertilizer × Straw
|
0.666
|
Temperate × Fertilizer × Straw
|
0.859
|
T3
|
Temperate
|
0.210
|
Fertilizer
|
0.640
|
Straw
|
0.851
|
Temperate × Fertilizer
|
0.222
|
Temperate × Straw
|
0.240
|
Fertilizer × Straw
|
0.448
|
Temperate × Fertilizer × Straw
|
0.259
|
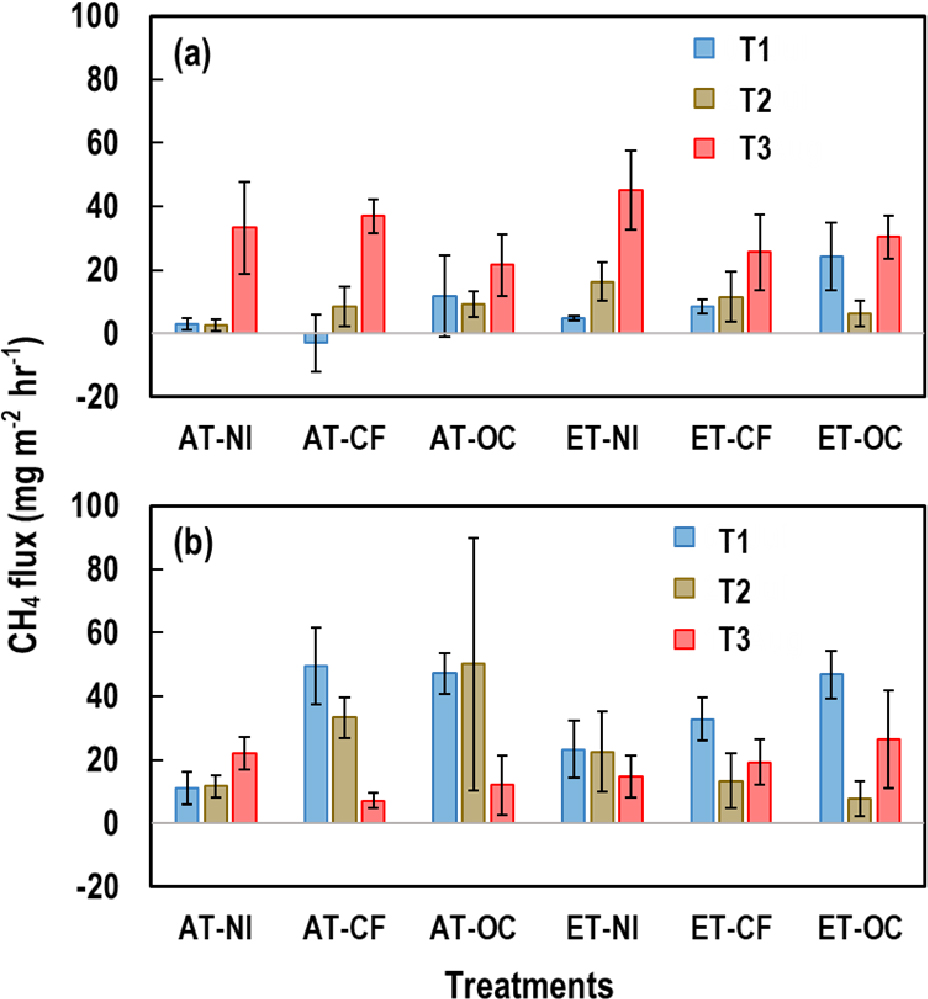
Fig. 2.
Total methane emission from both rice plants and soils through rice growing season across different temperature and fertilization treatments: (a) without straw input and (b) with straw input. AT and ET indicates ambient and elevated temperature, respectively, and NI, CF, and OC imply no fertilizer input, chemical fertilization, and organic fertilization with manure compost, respectively. Methane fluxes were before mid-season drainage (MSD) (T1), in the course of MSD (T2), and after MSD (T3). Values are the means of triplicate, and standard errors are depicted with the vertical bars.
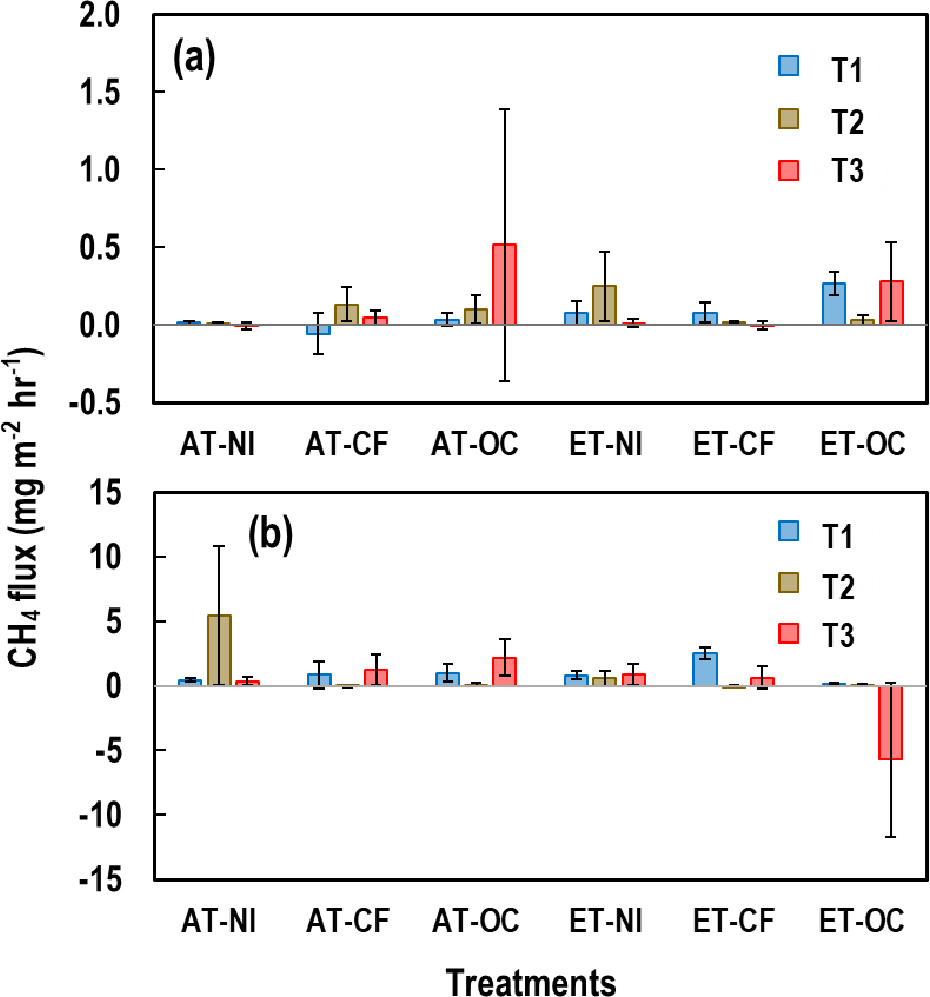
Fig. 3.
Methane emission directly from soil surface through rice growing season across different temperature and fertilization treatments: (a) without straw input and (b) with straw input. AT and ET indicates ambient and elevated temperature, respectively, and NI, CF, and OC imply no fertilizer input, chemical fertilization, and organic fertilization with manure compost, respectively. Methane fluxes were before mid-season drainage (MSD) (T1), in the course of MSD (T2), and after MSD (T3). Values are the means of triplicate, and standard errors are depicted with the vertical bars.
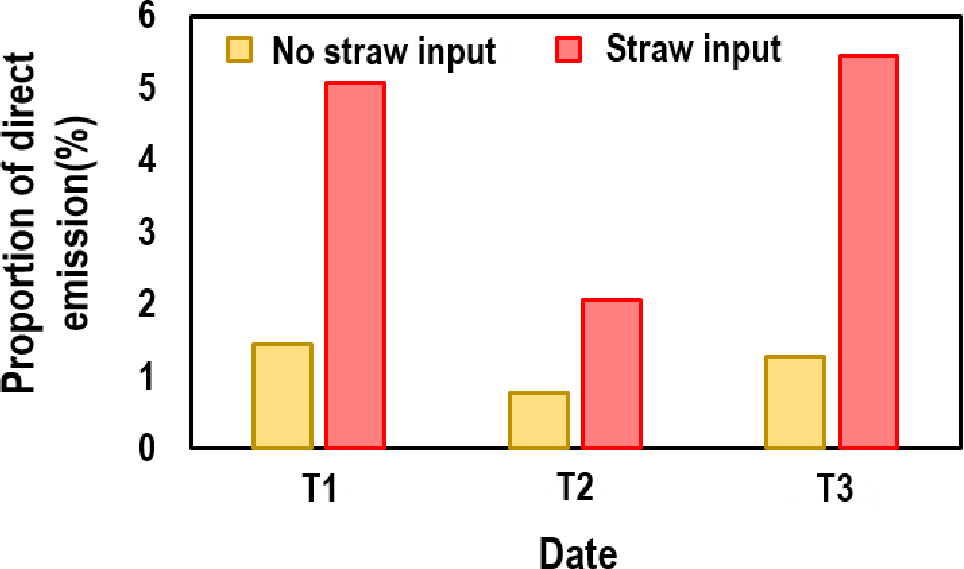
Fig. 4.
Portion of methane directly emitted from soil surface (MDS) to total methane emission from both rice plants and soils (MDPS) before mid-season drainage (MSD) (T1), in the course of MSD (T2), and after MSD (T3). The values are calculated using the mean MDS and MDPS across temperature and fertilization treatments, and thus further statistical analysis including standard error was not possible.
Table 3.
Probability values for the analysis of variance on the portion of soil direct methane emission to the total methane flux including rice and soil.
Treatments
|
Probability > F |
Temperate
|
0.774
|
Fertilizer
|
0.477
|
Straw
|
0.149
|
Time
|
0.501
|
Temperate × Fertilizer
|
0.853
|
Temperate × Straw
|
0.695
|
Temperate × Time
|
0.560
|
Fertilizer × Straw
|
0.580
|
Fertilizer × Time
|
0.535
|
Straw × Time
|
0.554
|
Temperate × Fertilizer × Straw
|
0.567
|
Temperate × Fertilizer × Time
|
0.889
|
Temperate × Straw × Time
|
0.790
|
Fertilizer × Straw × Time
|
0.610
|
Temperate × Fertilizer × Straw × Time
|
0.864
|
비록 유기질 비료와 퇴비 투입에 의해서도 상당한 수준의 유기탄소가 토양에 투입되었지만, 본 연구에서는 이에 의한 메탄 발생량 증가가 확인되지 않았다. 이는 유기질 비료에 의한 탄소 투입량은 볏짚의 20% 이하로 낮은 수준이었고, 퇴비의 유기탄소는 퇴비화 과정에서 난분해성으로 안정화되어 메탄 생성에 크게 기여하지 못한 것으로 판단된다 (Lim and Choi, 2014). 한편, 토양직접방출 메탄 플럭스가 중간낙수 후 볏짚 투입구 중 상승온도 조건에서 유기질 비료 + 퇴비가 처리된 조건에서 음의 값을 보였는데 (Fig. 3), 이는 혐기적 메탄 산화일 가능성으로 판단된다. 토양의 메탄 산화균이 메탄을 산화하는 과정에서 발생하는 전자가 전자수용체로 전달되어 전자수용체가 환원되는데 (Fan et al., 2022; Yoon et al., 2022), 다른 처리구와 달리 전자수용체 (e.g., Fe3+, Mn4+)를 함유한 유기질 비료 + 퇴비 처리구에서 이와 같은 혐기적 메탄 산화가 일어났을 것으로 추측된다 (Lim and Choi, 2014; Fan et al., 2021). Kong et al. (2019)의 연구 결과에 의하면, 화학비료 처리구에 비해 돈분 처리구에서 혐기적 메탄 산화에 의해 메탄 발생량이 오히려 감소하였다. 따라서, 분뇨와 퇴비 처리에 의한 혐기적 메탄 산화에 대한 추가적인 연구가 필요하다.
Conclusions
본 연구에서는 벼 생장기 동안 3회에 국한하여 토양직접방출 메탄을 측정하여 전체 벼 생육과정에 대한 대표성을 인정하기는 어렵지만, 조사기간에 방출된 전체 메탄의 약 1%가 토양에서 직접 배출되는 것으로 조사되었다. 하지만, 온도나 시비조건 보다는 볏짚 투입에 의해 토양직접방출 메탄 비율이 증가였는데, 특히 벼 통기조직이 미발달된 벼 생육초기인 중간낙수 전에 최대 5%까지 증가하였다. 따라서, 비록 벼 생육 초기에는 벼 통기조직을 통한 메탄 방출량은 적을 수 있지만, 볏짚 등 유기물이 투입된 조건에서는 토양에서 직접 배출되는 메탄의 기여도가 높을 것으로 예상된다.
Funding
This work was supported by the National Research Foundation of Korea (Project No. NRF-2022R1A2C1007402).
Conflict of Interest
The authors declare that they have no known competing financial interests or personal relationships that could have appeared to influence the work reported in this paper.
Author Contribution
Shin ES: Data curation, Writing-original draft, Baek N: Data curation, Writing-original draft, Park SW: Data curation, Pia HI: Data curation, Park HJ: Data curation, Writing-review & editing, Kim HY: Data curation, Writing-review & editing, Choi WJ: Supervision, Conceptualization, Writing-review & editing.
Data Availability
All data collected for this study are provided in Table or Figure.
Acknowledgements
The authors thank Chonnam National University for providing the TGCs facility.
References
Baek N, Park SW, Shin ES, Heo SB, Park HJ, Choi WJ. 2023. Changes in methane emission of Korean rice production systems for the last two decades and suggestions for methane mitigation. Korean J. Soil Sci. Fert. 56:199-208.
https://doi.org/10.7745/KJSSF.2023.56.2.19910.7745/KJSSF.2023.56.2.199Bhattacharyya P, Dash PK, Swain CK, Padhy SR, Roy KS, Neogi S, Berliner J, Adak T, Pokhare SS, Baig MJ, et al. 2019. Mechanism of plant mediated methane emission in tropical lowland rice. Sci. Total Environ. 651:84-92.
https://doi.org/10.1016/j.scitotenv.2018.09.14110.1016/j.scitotenv.2018.09.14130223222Denier van der Gon HAC, van Breemen N, Neue HU, Lantin RS, Aduna JB, Alberto MCR, Wassmann R. 1996. Release of entrapped methane from wetland rice fields upon soil drying. Global Biogeochem. Cycles 10:1-7.
https://doi.org/10.1029/95GB0346010.1029/95GB03460Fan L, Dippold MA, Thiel V, Ge T, Wu J, Kuzyakov Y, Dorodnikov M. 2022. Temperature sensitivity of anaerobic methane oxidation versus methanogenesis in paddy soil: Implications for the CH
4 balance under global warming. Global Change Biol. 28:654-664.
https://doi.org/10.1111/gcb.1593510.1111/gcb.1593534653297Fan L, Schneider D, Dippold MA, Poehlein A, Wu W, Gui H, Ge T, Wu J, Thiel V, Kuzyakov Y, et al. 2021. Active metabolic pathways of anaerobic methane oxidation in paddy soils. Soil Biol. Biochem. 156:108215.
https://doi.org/10.1016/j.soilbio.2021.10821510.1016/j.soilbio.2021.108215GIR (Greenhouse Gas Inventory and Research Center). 2022. National greenhouse gas inventory report of Korea. Ministry of Environment, Incheon, Korea.
Gwon HS, Choi EJ, Lee SI, Lee HS, Lee JM, Kang SS. 2022. Research review of methane emissions from Korean rice paddies. J. Clim. Change Res. 13:117-134.
https://doi.org/10.15531/KSCCR.2022.13.1.11710.15531/KSCCR.2022.13.1.117Gwon HS, Khan MI, Yoon YE, Lee YB, Kim PJ, Hwang HY. 2019. Unexpected higher decomposition of soil organic matter during cold fallow season in temperate rice paddy. Soil Tillage Res. 192:250-257.
https://doi.org/10.1016/j.still.2018.11.00910.1016/j.still.2018.11.009Jeong HC, Choi EJ, Lee JS, Kim GY, Lee SI. 2018. Comparison of CH
4 emission between auto chamber and manual chamber in the rice paddy. J. Clim. Change Res. 9:377-384.
https://doi.org/10.15531/KSCCR.2018.9.4.37710.15531/KSCCR.2018.9.4.377Ju O, Won TJ, Cho KR, Choi BR, Seo JS, Park IT, Kim GY. 2013. New estimates of CH
4 emission scaling factors by amount of rice straw applied from Korea paddy fields. Korean J. Environ. Agric. 32:179-184.
http://dx.doi.org/10.5338/KJEA.2013.32.3.17910.5338/KJEA.2013.32.3.179Kajiura M, Tokida T. 2021. Quantifying bubbling emission (ebullition) of methane from a rice paddy using high-time-resolution concentration data obtained during a closed-chamber measurement. J. Agric. Meteorol. 77:245-252.
https://doi.org/10.2480/agrmet.D-21-0002210.2480/agrmet.D-21-00022Kim GY, Park SI, Song BH, Shin YK. 2002. Emission characteristics of methane and nitrous oxide by management of water and nutrient in a rice paddy soil. Korean J. Environ. Agric. 21:136-143.
https://doi.org/10.5338/KJEA.2002.21.2.13610.5338/KJEA.2002.21.2.136Kim HY, Lim SS, Kwak JH, Lee DS, Lee SM, Ro HM, Choi WJ. 2011. Dry matter and nitrogen accumulation and partitioning in rice (
Oryza sativa L.) exposed to experimental warming with elevated CO
2. Plant Soil 342:59-71.
https://doi.org/10.1007/s11104-010-0665-y10.1007/s11104-010-0665-yKim J, Yoo G, Kim D, Ding W, Kang H. 2017. Combined application of biochar and slow-release fertilizer reduces methane emission but enhances rice yield by different mechanisms. Appl. Soil Ecol. 117:57-62.
https://doi.org/10.1016/j.apsoil.2017.05.00610.1016/j.apsoil.2017.05.006Kim S, Cho HS, Choi JS, Park KD, Jang JS, Kang S, Park JH, Kim MT, Kang IJ, Yang W. 2016. Changes in methane emissions from paddy under different tillage and cultivation methods. Korean J. Crop Sci. 61:251-256.
https://doi.org/10.7740/kjcs.2016.61.4.25110.7740/kjcs.2016.61.4.251Kong D, Li S, Jin Y, Wu S, Chen J, Hu T, Wang H, Liu S, Zou J. 2019. Linking methane emissions to methanogenic and methanotrophic communities under different fertilization strategies in rice paddies. Geoderma 347:233-243.
https://doi.org/10.1016/j.geoderma.2019.04.00810.1016/j.geoderma.2019.04.008Lee JH, Lee JG, Jeong ST, Gwon HS, Kim PJ, Kim GW. 2020a. Straw recycling in rice paddy: Trade-off between greenhouse gas emission and soil carbon stock increase. Soil Tillage Res. 199:104598.
https://doi.org/10.1016/j.still.2020.10459810.1016/j.still.2020.104598Lee JH, Park MH, Song HJ, Kim PJ. 2020b. Unexpected high reduction of methane emission via short-term aerobic pre-digestion of green manured soils before flooding in rice paddy. Sci. Total Environ. 711:134641.
https://doi.org/10.1016/j.scitotenv.2019.13464110.1016/j.scitotenv.2019.13464131822416Lee JM, Jeong HC, Lee HS, Park HR, Kim GS, Lee SI. 2023. Effects of water management practices on methane emissions and rice yields in East Asian paddy fields: A regional-scale meta-analysis. Korean J. Soil Sci. Fert. 56:313-324.
https://doi.org/10.7745/KJSSF.2023.56.4.31310.7745/KJSSF.2023.56.4.313Lim SS, Choi WJ. 2014. Changes in microbial biomass, CH
4 and CO
2 emissions, and soil carbon content by fly ash co-applied with organic inputs with contrasting substrate quality under changing water regimes. Soil Biol. Biochem. 68:494-502.
https://doi.org/10.1016/j.soilbio.2013.10.02710.1016/j.soilbio.2013.10.027Malyan SK, Bhatia A, Kumar A, Gupta DK, Singh R, Kumar SS, Tomer R, Kumar O, Jain N. 2016. Methane production, oxidation and mitigation: A mechanistic understanding and comprehensive evaluation of influencing factors. Sci. Total Environ. 572:874-896.
https://doi.org/10.1016/j.scitotenv.2016.07.18210.1016/j.scitotenv.2016.07.18227575427Minamikawa K, Tokida T, Sudo S, Padre A, Yagi K. 2015. Guidelines for measuring CH
4 and N
2O emissions from rice paddies by a manually operated closed chamber method. National Institute for Agro-Environmental Sciences, Tsukuba, Japan.
NAAS (National Academy of Agricultural Science). 2013. Cultivation methods to reduce greenhouse gases. RDA, Jeonju, Korea.
Nam HS, Kwak JH, Lim SS, Choi WJ, Lee SI, Lee DS, Lee KS, Kim HY, Lee SM, Matsushima M. 2013. Fertilizer N uptake of paddy rice in two soils with different fertility under experimental warming with elevated CO
2. Plant Soil 369:563-575.
https://doi.org/10.1007/s11104-013-1598-z10.1007/s11104-013-1598-zPark HJ, Lim SS, Kwak JH, Lee KS, Yang HI, Kim HY, Lee SM, Choi WJ. 2020. Biomass, chemical composition, and microbial decomposability of rice root and straw produced under co-elevated CO
2 and temperature. Biol. Fertil. Soils 56:991-1005.
https://doi.org/10.1007/s00374-020-01471-y10.1007/s00374-020-01471-ySass RL, Fisher FM, Harcombe PA, Turner FT. 1990. Methane production and emission in a Texas rice field. Global Biogeochem. Cycles 4:47-68.
https://doi.org/10.1029/GB004i001p0004710.1029/GB004i001p00047Seo YJ, Park JH, Kim CY, Kim JS, Cho DH, Choi SY, Park SD, Jung HC, Lee DB, Kim KS, et al. 2011. Effects of soil types on methane gas emission in paddy during rice cultivation. Korean J. Soil Sci. Fert. 44:1220-1225.
https://doi.org/10.7745/KJSSF.2011.44.6.122010.7745/KJSSF.2011.44.6.1220Tokida T, Cheng W, Adachi M, Matsunami T, Nakamura H, Okada M, Hasegawa T. 2013. The contribution of entrapped gas bubbles to the soil methane pool and their role in methane emission from rice paddy soil in free-air [CO
2] enrichment and soil warming experiments. Plant Soil 364:131-143.
https://doi.org/10.1007/s11104-012-1356-710.1007/s11104-012-1356-7Tokida T, Miyazaki T, Mizoguchi M, Nagata F, Takakai F, Kagemoto A, Hatano R. 2007. Falling atmospheric pressure as a trigger for methane ebullition from peatland. Global Biogeochem. Cycles 21:GB2003.
https://doi.org/10.1029/2006GB00279010.1029/2006GB002790Wang C, Wang W, Sardans J, Singla A, Zeng C, Lai DYF, Peñuelas J. 2019. Effects of steel slag and biochar amendments on CO
2, CH
4, and N
2O flux, and rice productivity in a subtropical Chinese paddy field. Environ. Geochem. Health 41:1419-1431.
https://doi.org/10.1007/s10653-018-0224-710.1007/s10653-018-0224-730535544Wang J, Zhang X, Xiong Z, Khalil MAK, Zhao X, Xie Y, Xing G. 2012. Methane emissions from a rice agroecosystem in South China: Effects of water regime, straw incorporation and nitrogen fertilizer. Nutr. Cycling Agroecosyst. 93:103-112.
https://doi.org/10.1007/s10705-012-9503-310.1007/s10705-012-9503-3Yoon S, Lee J, Lee Y, An H, Jung KY, Oh SH, Lee CH, Kim SY. 2022. Long-term inorganic and organic fertilizations affect CH
4 oxidation potential and methanotrophic community structure in paddy soils. Korean J. Soil Sci. Fert. 55:475-487.
https://doi.org/10.7745/KJSSF.2022.55.4.47510.7745/KJSSF.2022.55.4.475Zhao Z, Yue Y, Sha Z, Li C, Deng J, Zhang H, Gao M, Cao L. 2015. Assessing impacts of alternative fertilizer management practices on both nitrogen loading and greenhouse gas emissions in rice cultivation. Atmos. Environ. 119:393-401.
https://doi.org/10.1016/j.atmosenv.2015.08.06010.1016/j.atmosenv.2015.08.060